"Above V1 we are going." Period, end of discussion. You might want to add a caveat to that statement: "Unless the airplane will not fly."
— James Albright
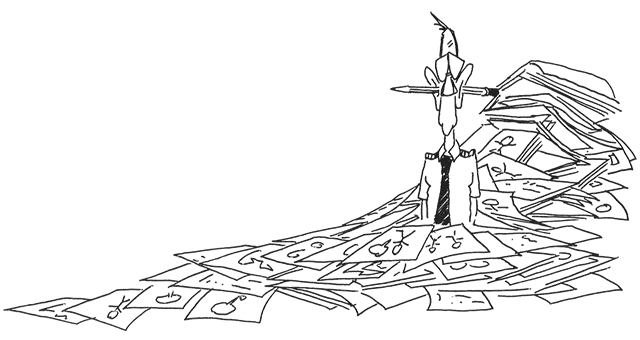
Updated:
2017-09-15
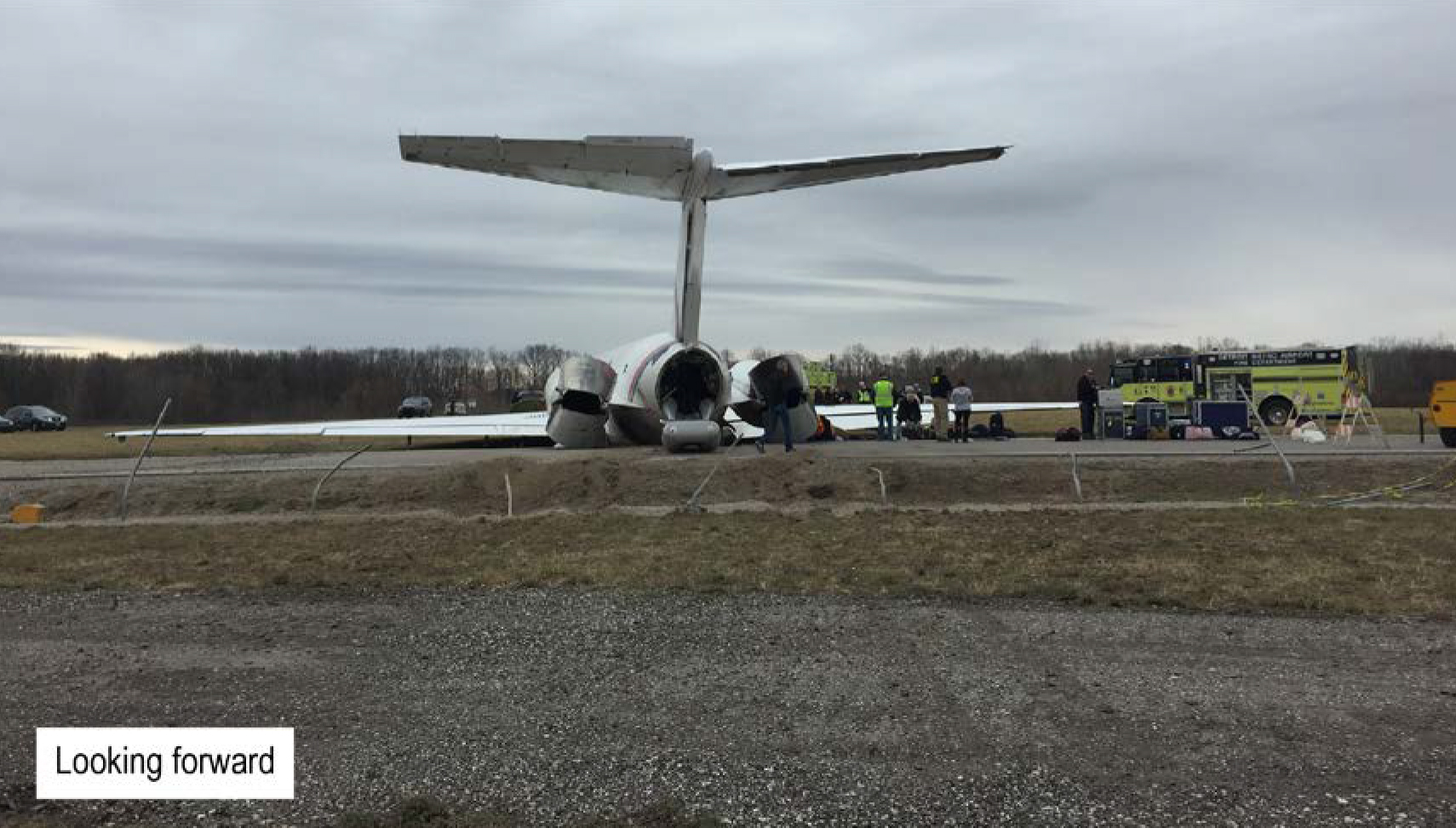
Rear view of the airplane wreckage, NTSB AAR 19/01, figure 2
As pilots we hope (and endeavor) to never find ourselves the subject of an aircraft accident investigation, but if we are, we hope that all our actions in hindsight were the right actions. This is just such an example.
The accident was caused by a flaw in the aircraft's design that had not been foreseen. The captain was the pilot flying and the first officer was a check airman. I don't know if this impacted the situation but both appeared to be operating by the book, according to the company's Standard Operating Procedures (SOPs). Perhaps we can take a lesson from this. If both pilots are operating as if the other pilot is keeping a close eye on them or in a manner to demonstrate to the other pilot only the best example, we will have set ourselves up for success in even the most trying of circumstances.
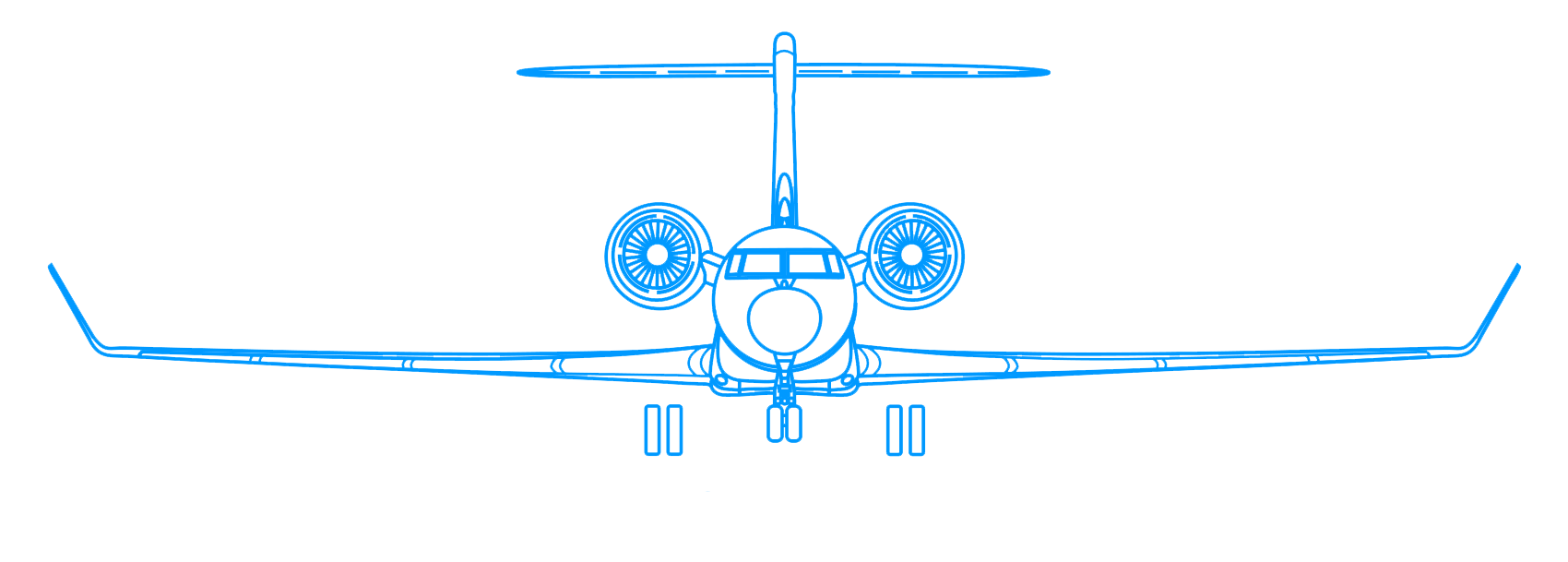
1
Accident report
- Date: 8 March 2017
- Time: 14:52
- Type: McDonnell Douglas MD-83
- Operator: Ameristar Jet Charter
- Registration: N786TW
- Fatalities: 0 of 6 crew, 0 of 110 passengers
- Aircraft fate: Damaged
- Phase: Takeoff
- Airport (departure): Detroit-Willow Run Airport, MI (KYIP), USA
- Airport (arrival): Washington-Dulles International Airport, DC (KIAD), USA
2
Narrative
Everything before, during, and after this accident was by the book, as far as the pilots were concerned. There may have been issues with the evacuation that were overcome, but our focus here is on pilot procedures.
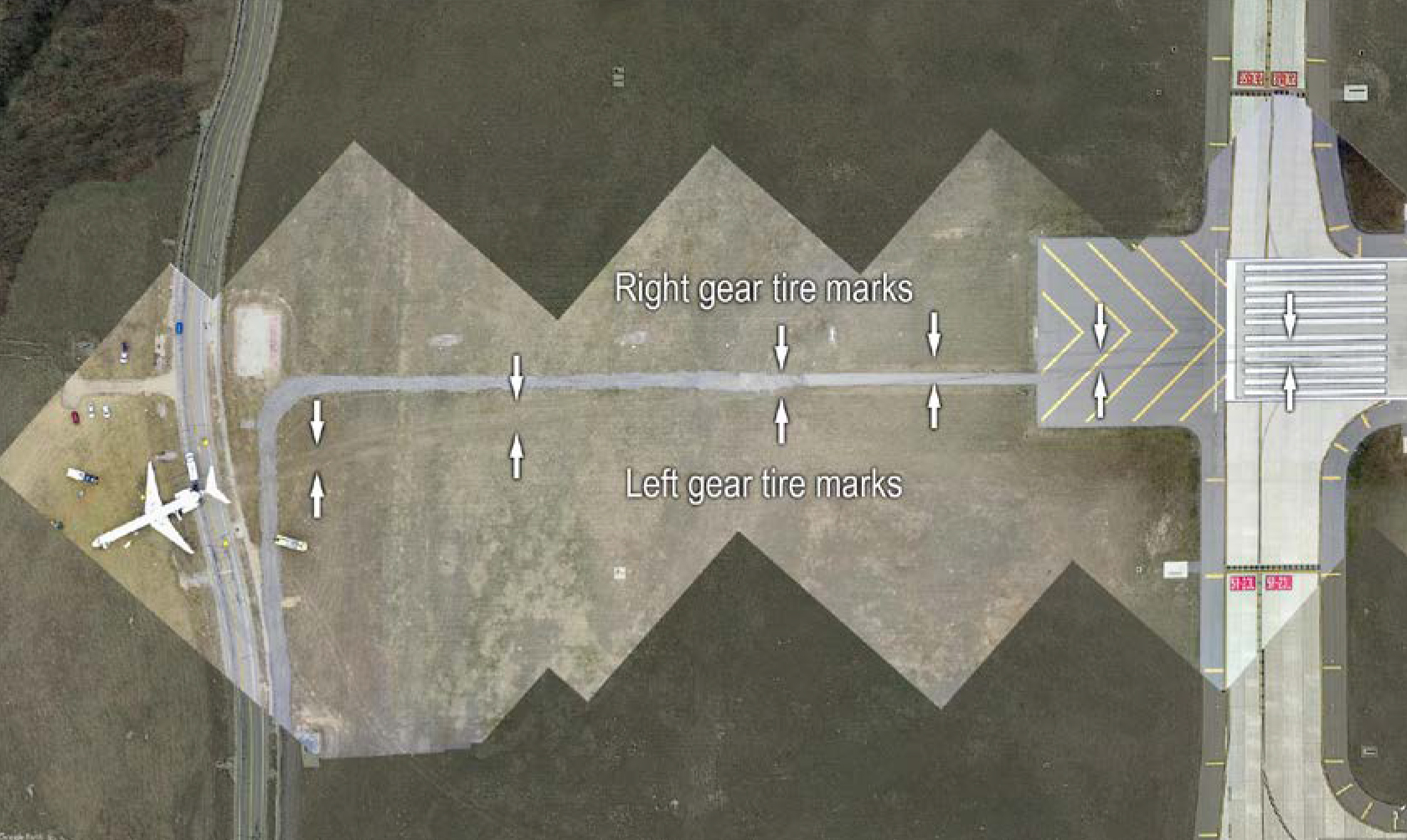
Orthomosaic image of the airplane's path from the end of runway 23L to its final location, NTSB AAR 19/01, figure 1.
- On March 8, 2017, about 1452 eastern standard time (EST), Ameristar Air Cargo, Inc., dba Ameristar Charters, flight 9363, a Boeing MD-83 airplane, N786TW, overran the departure end of runway 23L at Willow Run Airport (YIP), Ypsilanti, Michigan, after the captain executed a rejected takeoff. The 110 passengers and 6 flight crewmembers (two airline transport pilots [ATPs] and four flight attendants) evacuated the airplane via emergency escape slides. One passenger received a minor injury, and the airplane sustained substantial damage. The airplane was operated under the provisions of Title 14 Code of Federal Regulations (CFR) Part 121 as an on-demand charter flight and was destined for Washington Dulles International Airport, Dulles, Virginia. Visual flight rules (VFR) conditions prevailed at YIP at the time of the accident.
- The airplane had been parked at YIP for 2 days before the accident on a ramp area east of a large (about 0.25-mile long) hangar. About 1139 on the morning of the accident, high wind from the west resulted in a power outage at the airport that affected some weather observing equipment and prompted the air traffic control tower (ATCT) personnel to evacuate the tower. According to Federal Aviation Administration (FAA) records, the ATCT issued a notification at 1217 to advise that the airport had no air traffic control (ATC) services (referred to as “ATC Zero”).
- The flight crew first powered up the airplane about 1236. They followed company procedures for operations at nontowered airports and used a cell phone to obtain updated weather information and ATC clearances. The captain, seated in the left seat, was the pilot flying (PF), and the check airman in the right seat (who was providing differences training to the captain) was the pilot monitoring (PM). The captain performed the preflight walkaround inspection of the airplane and noticed no anomalies.
- During their preflight planning, the flight crewmembers considered the high gusting wind when discussing the V-speed calculations. During a postaccident interview, the check airman stated that they chose to use a maximum thrust takeoff, which was their normal procedure. According to the flight crew’s completed takeoff speed card recovered from the airplane, they calculated the V-speeds as follows: V1 (takeoff decision speed) was 139 kts, VR (rotation speed) was 142 kts, and V2 (minimum takeoff safety speed) was 150 kts. He also stated during the interview that the wind was “pretty gusty,” so they agreed to increase the rotation speed about 5 kts. The cockpit voice recorder (CVR) transcript indicated that the check airman advised the captain to “delay rotation until at least V2 . . . wait for me to call it.” The captain later confirmed their plan during his briefing, affirming to the check airman that “we are going to delay our rotation because of the gusty, strong gusty winds.”
- The captain’s briefing also considered wind in the event of an emergency; according to the CVR transcript, he told the check airman to
- While performing all their predeparture procedures and checklist items, neither pilot observed any anomalies with the airplane. The check airman performed the flight control checks during taxi and felt nothing unusual when he moved the control column forward and aft.
- The flight crew positioned the airplane for departure from runway 23L, and, at 1451:12, the check airman called for the captain to begin the takeoff roll. At 1451:55, the check airman called “V1.” Six seconds later (at 1452:01), he called “rotate,” followed 3 seconds later (at 1452:04) by “V2.” At 1452:05, the captain said, “hey, what’s goin’ on?” and, 3 seconds later, “abort.” The check airman stated, “no, not above…” and then “…don’t abort above V1 like that,” and the captain replied, “it wasn’t flying.”
- At 1452:23, the CVR captured sounds consistent with the airplane’s excursion from the paved surface. The airplane exited the paved surface off the departure end of runway 23L (including a 200 ft blast pad) and traveled about 950 ft across the grassy part of the runway safety area (RSA) before striking the airport perimeter fence and a raised, paved road before coming to a stop. The airplane came to rest on the fuselage belly with the tail on the road, about 1,150 ft west-southwest of the end of the runway.
- During a postaccident interview, the captain recalled that, when he began a normal rotation of the airplane at the “rotate” call, it did not rotate, so he applied more back pressure. (During a normal takeoff at rotation speed, pulling the control column aft results in trailing edge up [TEU] elevator movement to produce airplane-nose-up pitch) The captain said the control column was not quite to the physical limit of aft movement but was “further back than for a normal rotation.” Both pilots stated in interviews that, after the captain called for the rejected takeoff, they applied maximum braking, but the airplane went off the end of the runway.
- Initial examination of the airplane found that the airplane’s right elevator was jammed in a trailing edge down (TED) position and could not be moved when manipulated by hand
really keep an eye out on what our airspeed is doing today, ahm, in the event of an engine fire or failure at or after V1, we’re going to continue the takeoff . . . . [I]f you get any kind of a [windshear] warning, it’s gonna be max thrust, ah, all the way to the firewall thrust, if necessary . . . we’ll fly out of the shear, back me up on the, ah, airspeed calls.
Source: NTSB AAR 19/01, ¶1.1
- A review of airplane flight logs from October 2016 through March 2017 revealed no flight control discrepancies. Both right and left elevators were last lubricated on December 30, 2016.
- A records review that focused on elevator and stabilizer entries found that a C-check, including all nonroutine work cards, was completed in August 2015.9 The C-check requirements included detailed visual inspections of the horizontal stabilizer and elevator skins, internal structures, attachments, and actuation mechanisms. The inspection was completed with no nonroutine work cards generated for these components. A corrosion control inspection reported no findings of corrosion.
- The previous C-check, completed in July 2013, included seven nonroutine work cards for discrepancies found on both elevators and tabs. Four of the nonroutine items involved hail dents that required reinspections. A special inspection task was created for the 500-hour inspection of the hail dents for each elevator and tab. The right elevator float tab was found to be delaminated, which required a more restrictive 300-hour repetitive inspection per the Structural Repair Manual. A 300-hour repetitive inspection was not found; the inspection was included in the 500-hour repetitive inspection. The airplane had flown 611.4 flight hours since the July 2013 C-check when the delamination was discovered.
Source: NTSB AAR 19/01, ¶1.3.1.1
3
Analysis
I've flown aircraft with similar flight controls and it can be confusing for someone who grew up on simple control systems where a cable from the stick or yoke goes directly to the control surface, and then progressed to a hydraulic or fly-by-wire system. In a nutshell, the yoke is controlled to a smaller surface on the elevator, rudder, or aileron. The yoke moves the smaller control surface opposite the intended direction to provide an aerodynamic force to the large control surface. It was an ingenious way to control large aircraft in the days before hydraulic boost or fully hydraulic flight controls.
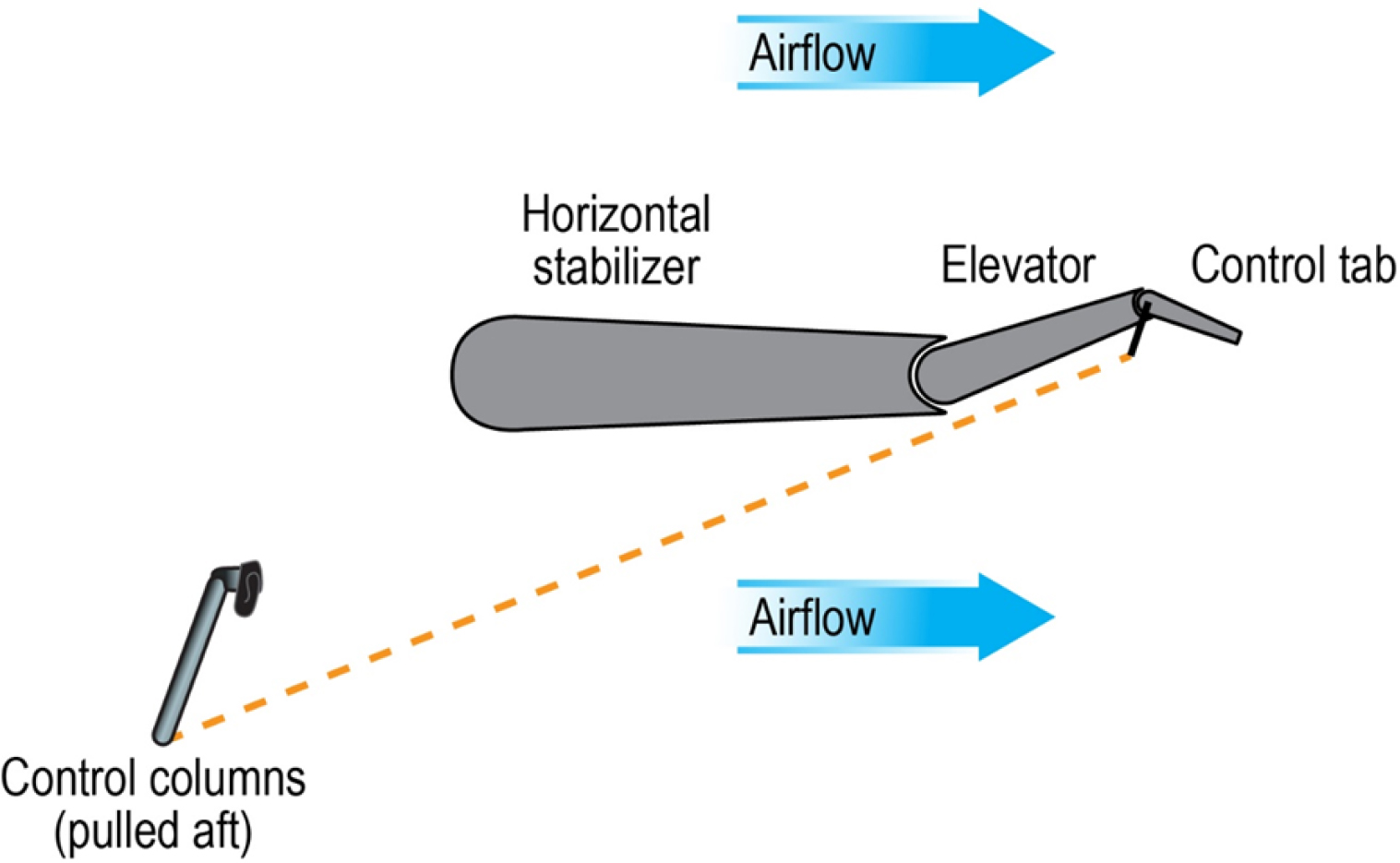
Right elevator and relative locations of control, geared, and antifloat tabs, NTSB AAR 19/01, figure 3
Elevator control
- The airplane is a t-tail design, such that the elevators and horizontal stabilizer are attached near the top of the vertical stabilizer about 30 ft above ground level (agl). The left and right elevators are attached by hinges to the rear spar of the horizontal stabilizer, and each is equipped with control, geared, and antifloat tabs attached to the trailing edge. Each elevator can travel between 27° TEU and 16.5° TED between mechanical stops mounted on the horizontal stabilizer. (A stop arm on each elevator contacts the mechanical stops to limit elevator travel, and a torsion bar distributes the increased loading.)
- Each elevator is also equipped with a damper designed to prevent elevator flutter during flight and dampen rapid movement of the elevator during gusty wind when the airplane is on the ground. When the airplane is parked, each elevator is free to move independently within the confines of the mechanical stops if acted upon by an external force, such as wind or manipulation by maintenance personnel. The elevator system (by design) has no gust lock, and the elevators are not interconnected.
- Elevator control is accomplished via the trailing-edge elevator control tabs, which are mechanically connected to and directly controlled by the cockpit control column. During takeoff (at VR or higher) and during flight, when a pilot provides aft or forward control column input to command a change in airplane pitch, the elevator control tabs mechanically deflect, and the resultant aerodynamic forces on the deflected control tabs move the elevator surfaces to produce the change in airplane pitch. For example, when a pilot pulls the control column aft to command airplane nose-up pitch (such as during rotation), the control tabs respond by deflecting TED, and the resultant aerodynamic forces move the elevators TEU.
- Elevator geared tabs, which mechanically deflect in response to elevator movements, are attached to the horizontal stabilizer through a system of drive linkages. The geared tab drive linkage consists of a pushrod that is attached to the horizontal stabilizer spar by means of an actuating crank and links. The antifloat tabs are connected to the horizontal stabilizer through mechanical linkages and deflect based on stabilizer position to prevent down-float of the elevator when the horizontal stabilizer position exceeds certain criteria.
Source: NTSB AAR 19/01, ¶1.3.2.1
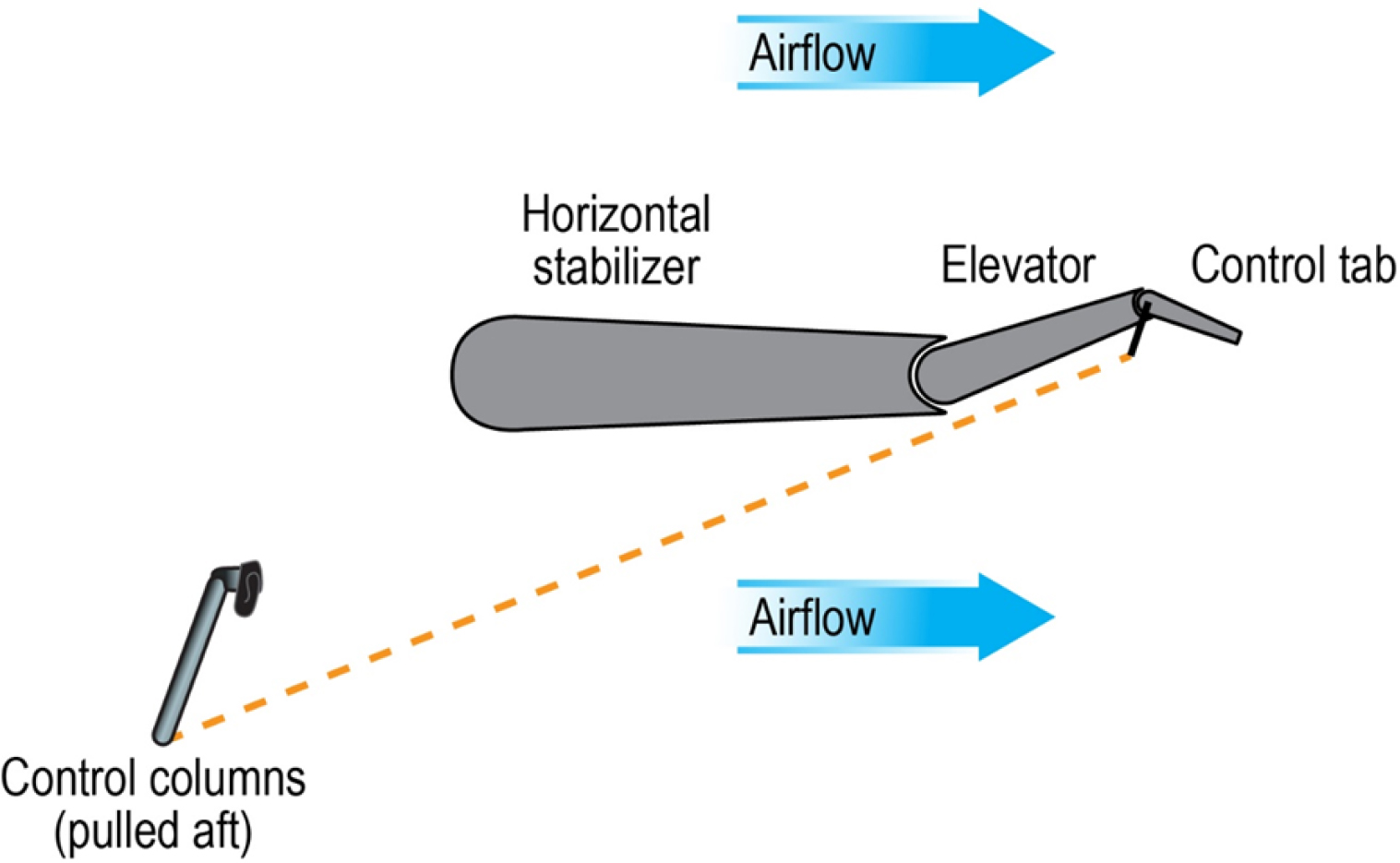
Simplified diagram of elevator control, NTSB AAR 19/01, figure 4
Damage to elevator
- During an on-scene postaccident examination, investigators established flight control continuity for the elevator system by exercising the cockpit control columns through their full range to the control column stops in both the aircraft nose-up and aircraft nose-down directions. The left and right elevator control tabs responded with movement in the appropriate direction. Investigators noted that the control columns in some positions did not move smoothly, and there were tactile and audible indications of control cable friction under the cockpit floor. Further examination revealed airplane structural damage in an area where the cables were routed; the cables were riding on the damaged structure.
Source: NTSB AAR 19/01
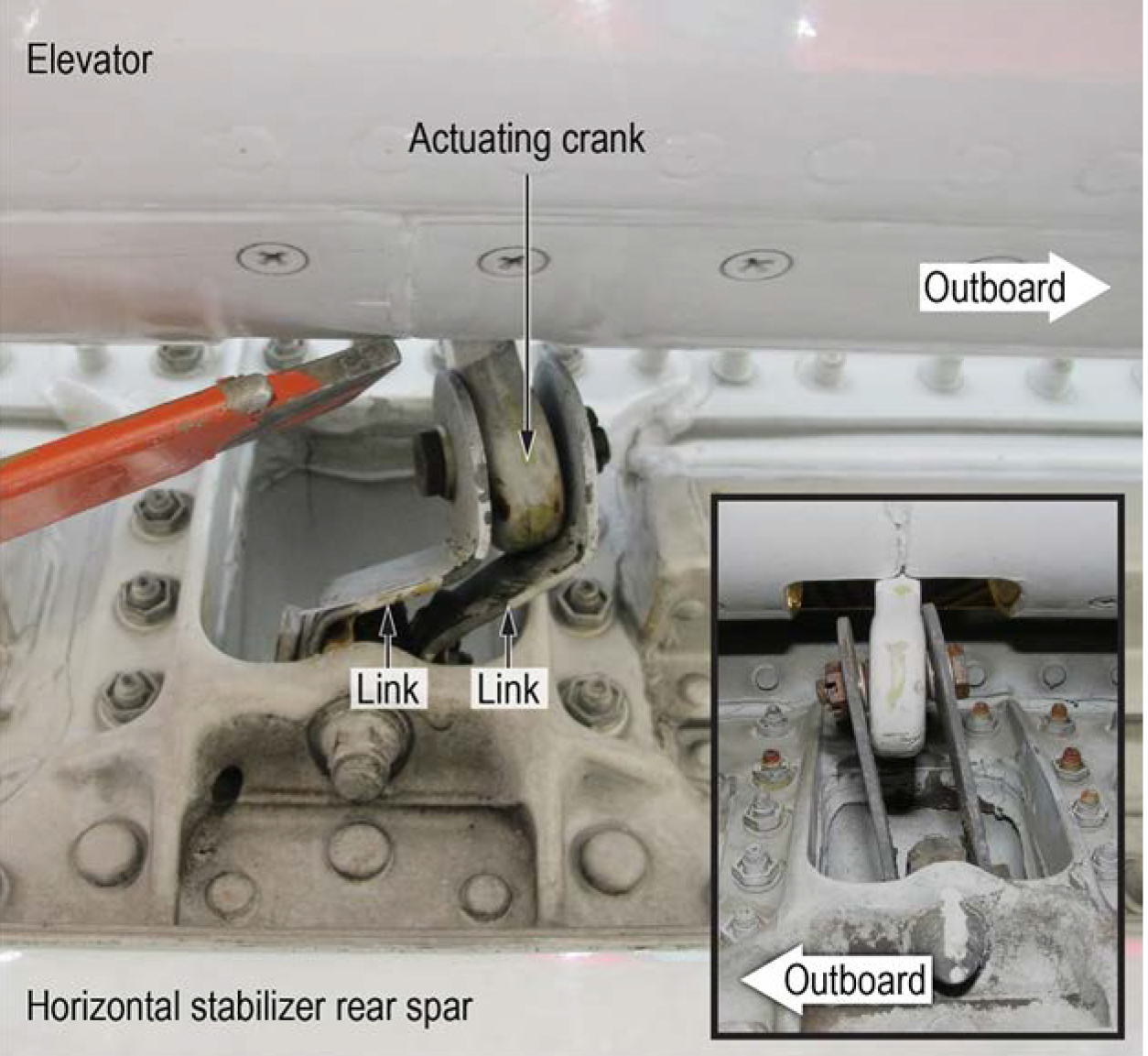
View of damaged elevator geared tab links and actuating crank in an overcenter position. Inset shows undamaged exemplar, NTSB AAR 19/01, figure 6
Note: the orange bar is not a part of the airplane and was used as a pointer.
- Using a lift to access the elevators, investigators found that the airplane’s right elevator was jammed in a TED position and could not be moved when manipulated by hand. Examination found that the inboard actuating crank for the right elevator’s geared tab was bent outboard, and its links were bent. Further, the actuating crank and links were found locked overcenter beyond their normal range of travel
Source: NTSB AAR 19/01
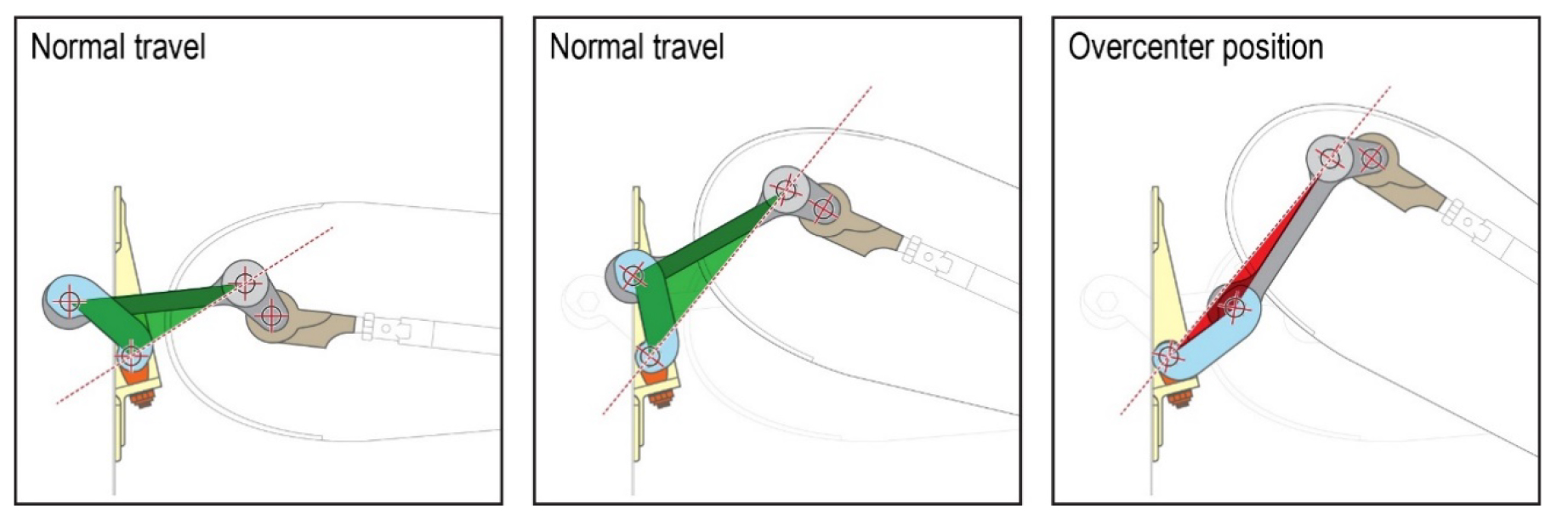
Diagram showing relative positions of the links (blue) and actuating crank (gray) when moving within their normal range of travel (in green) and when locked overcenter after having moved beyond their normal range of travel (in red), NTSB AAR 19/01, figure 7
- Examination of the left elevator found that it moved freely when manipulated by hand, and the left geared tab and linkages moved normally. When the left elevator surface was manipulated through its full range of motion (between the TED and TEU stops), the inboard actuating crank and links for the left geared tab moved within their normal travel range, and their relative positions never neared overcenter. Both elevators (including torsion bars, stop arms, and trailing-edge tabs), dampers, and boost cylinders were removed from the airplane for further examination and testing.
- The airworthiness regulation effective at the time the airplane was originally certificated specified a ground gust value of 88 ft per second (about 52 kts); it was revised in 1997 to increase the ground gust speed to 65 kts. Boeing stated in its submission to the investigation that the MD-80 series airplanes (which include the MD-83) were designed to withstand a 65 kt horizontal ground gust. According to Boeing, in the history of this elevator design (which exists on all Boeing DC-9/MD-80 series and 717 model airplanes), this accident was the first notification that Boeing had received of an elevator jam occurring on an airplane exposed to ground gusts lower than 65 kts. Boeing noted that the elevator design first entered service in 1965 on the then-Douglas DC-9 airplane.
Source: NTSB AAR 19/01
Weather
On the morning of the accident, the METAR issued at 1153 was the last report for which complete ASOS data with LAWRS observer augmentation were available. It contained the following information:
Wind from 260° at 35 kts with gusts to 50 kts, visibility 10 miles or greater, sky clear below 12,000 ft agl, temperature 11°C, dew point -11°C, altimeter setting of 29.81 inches of mercury with remarks that the station had a precipitation discriminator, a peak wind from 260° of 55 kts occurred at 1139, sea level pressure was 1009.5 hectopascals, temperature 10.6°C, and dew point -10.6°C.
Source: NTSB AAR 19/01, ¶1.4.2.2
The NTSB and Boeing conducted a fascinating study of the local wind effects from a nearby hangar showed that a 55 knot gust over the hangar could have produce a horizontal gust of over 58 knots over the airplane. This is still below the design limit of the elevator.
Elevator performance
- FDR data showed that, from the time that the accident airplane was first powered up (about 1236) on the day of the accident, repositioned for loading (about 1242 to 1249), and then prepared and taxied for takeoff (beginning about 1435), the left elevator moved in the wind to various positions between about -16° TED and 27° TEU, but the right elevator did not move and remained about -16° TED.
- As described in section 1.1, before takeoff, the flight crew determined V1, VR, and V2 to be 139 kts, 150 kts, and 150 kts, respectively. (They calculated VR to be 142 kts but chose to increase it about 5 kts because of the strong, gusty wind conditions.) The performance study determined that, during the attempted takeoff, at 1451:56 (about 1 second after the check airman called “V1”), the airplane was at an airspeed of about 138 kts, a slight input on the elevator control column was applied, the airplane’s left elevator moved from -15° TED to -13° TED, and the right elevator did not move, remaining about -16° TED.
- Five seconds later, at 1452:01 (when the check airman called “rotate”), the airspeed was about 151 kts, and a large control column input was applied over the next 8 seconds. The left elevator moved to about 15° TEU, and the right elevator remained about -16° TED until the last 3 seconds of maximum control column input, at which time the right elevator moved to about -13° TED. The airplane did not respond in pitch and did not rotate.
Source: NTSB AAR 19/01, ¶1.10.1.1
Elevator tests
Investigators constructed a rig with the elevator assembly to see what happens when it was subjected to wind gusts. They wanted to find out if a gust could have caused the elevator to go overcenter. They discovered that even with gusts up to 75 knots, this would not happen if the elevator began the test with the elevator not moving (static). But if the elevator started from a neutral position, a 65 knot gust or greater would cause it to go overcenter. If the elevator started full TEU, a 60 knot gust would also cause it to go overcenter.
- For each static load test, the elevator was positioned such that the stop arm was resting on the TED stop when the load was applied. Tests that simulated gust loads of 25, 55, and 75 kts (the only speeds tested for the static condition) both with and without boost cylinder activation revealed that the applied loads resulted in torsion bar rotation and elevator position travel beyond the nominal unloaded TED stop position of 16.5°. Each successive increase in load resulted in increased torsion bar rotation and elevator surface deflection, and the tests performed with the boost cylinder activation produced comparatively greater rotation and deflection for each load than the tests without the activation. None of the static tests resulted in the geared tab linkage becoming overcenter.
- For each dynamic test, the load was applied to the elevator; the elevator was then raised to either a neutral or full-TEU position (using a forklift and lifting straps) before it was released (using a quick-release mechanism). Releasing the lifted elevator from either the neutral or full-TEU position allowed it to travel downward and dynamically contact the TED stop.
- A series of dynamic tests without the boost cylinder activation and with the elevator starting in either a neutral or full-TEU position did not result in the geared tab linkage becoming overcenter for simulated gust loads of 25 and 55 kts. For the 60-kt simulated gust load, the linkage became overcenter for only the full-TEU initial elevator position test (see table 2). For the 65-, 70-, and 75-kt simulated gust loads, the linkage became overcenter for the neutral initial elevator position tests (the full-TEU initial position was not tested for these loads).
Source: NTSB AAR 19/01, ¶1.10.4.1
Based on the results of the testing conducted, gust loads between 55 and 60 kts could result in an overcenter condition of the geared tab linkage if the elevator traveled from a full-TEU position to the TED stop. Thus, the NTSB concludes that, although the reported wind at the airport did not exceed the horizontal ground gust limits specified for transport-category airplanes, the effects of the large hangar near the accident airplane’s parked location resulted in a turbulent windflow with vertical components that imposed dynamic loads on the elevator system sufficient to jam the right elevator.
Source: NTSB AAR 19/01, ¶2.3.3
Parking procedures in gusty conditions
- An amplified normal checklist in Ameristar’s Boeing DC-9 (Boeing MD-83) Aircraft Operating Manual (AOM) (volume 1, page 1.2-2) contained the following caution:
- Ameristar’s Boeing DC-9 (Boeing MD-83) AMM contained the following warning and caution under “Parking: General Procedures”:
Airplanes that are exposed to high-sustained winds, or wind gusts, greater than 75 [mph] (approximately 65 [kts]) are susceptible to elevator damage and/or jamming. In accordance with the . . . [AMM], airplanes suspected to have been exposed to these conditions must have visual and physical inspections (moving surfaces by hand) of all flight controls and operational check of these [systems].
If wind gusts are expected to exceed 69 [mph] (60 [kts]), aircraft should be headed into wind to prevent structural damage to primary control surfaces.
CAUTION: If there is any possibility that [the] aircraft has been subjected to winds in excess of 75 [mph] (65 [kts]), and aircraft has not been headed into wind or wind direction changed during parking, perform visual and physical inspections (moving the surfaces by hand) of all flight controls and an operational check of these systems.
Source: NTSB AAR 19/01, ¶1.11.3.1
Ameristar’s AMM for the Boeing MD-83 included a warning that the airplane must be parked headed into the wind if gusts were expected to exceed 60 kts and a caution that a visual and physical inspection of all flight control surfaces was required if the airplane was subjected to wind exceeding 65 kts. However, none of the recorded or forecasted wind at YIP exceeded these limits during the time that the airplane was parked on the ramp (the highest reported wind gust was 55 kts and the highest forecasted gust was 48 kts). Thus, the NTSB concludes that, based on the available wind data for YIP, the flight crew was not required to perform high-wind parking procedures or request flight control inspections from maintenance personnel.
Source: NTSB AAR 19/01, ¶2.2.1.1
The control tabs are the only parts of the elevator system that are connected to the control column, and the control tabs were not damaged. Thus, the NTSB concludes that the flight crew’s preflight inspection and control check during taxi, which were performed in accordance with the procedures specified in the AOM, could not have detected the overcenter position of the right elevator geared tab’s linkage or the resultant jammed elevator condition.
Source: NTSB AAR 19/01, ¶2.2.1.2
Crew coordination
- Crew coordination during takeoff is essential to managing one of the most critical phases of a flight. Effective crew coordination and performance depend on the flight crewmembers having a shared mental model of each task; such a mental model, in turn, is founded on effective standard operating procedures (SOPs) (FAA 2017b). Flight crew adherence to SOPs during a takeoff, including maintaining the defined roles of PF and PM, is of paramount importance to flight safety (FAA 2017b).
- Although Ameristar’s procedures for a rejected takeoff clearly establish that the responsibility for the go/no-go decision is exclusively that of the captain, in this flight, the PM was also a check airman providing airplane differences instruction to the captain trainee; thus, the check airman was the PIC of the flight. This increased the potential for confusion as to who was truly responsible for the go/no-go decision during an anomalous situation. Instructors typically have more experience in the airplane than the pilot receiving instruction (as was the case with this crew) and are primed to assume control should the trainee’s actions pose a risk to the flight. Although the check airman instinctively reached toward the control column after the captain’s “abort” callout (and stated to the captain that they should not reject a takeoff after V1), the check airman did not take control of the airplane but rather observed that the captain had initiated the rejected takeoff procedures and then took action to assist the captain in executing those procedures.
- The flight crew’s coordinated performance around the moment that the captain rejected the takeoff showed that both pilots had a shared mental model of their responsibilities. By adhering to SOPs—rather than reacting and taking control of the airplane from the captain trainee—the check airman demonstrated disciplined restraint in a challenging situation. Had the check airman simply reacted and assumed control of the airplane after the captain decided to reject, the results could have been catastrophic if such action were to further delay the deceleration (at best) or to try to continue the takeoff in an airplane that was incapable of flight. Thus, the NTSB concludes that the check airman’s disciplined adherence to company SOPs after the captain called for the rejected takeoff likely prevented further damage to the airplane and reduced the possibility of serious or fatal injuries to the crew and passengers.
Source: NTSB AAR 19/01, ¶2.2.3
4
Cause
The NTSB determines that the probable cause of this accident was the jammed condition of the airplane’s right elevator, which resulted from exposure to localized, dynamic wind while the airplane was parked and rendered the airplane unable to rotate during takeoff. Contributing to the accident were (1) the effect of a large structure on the gusting surface wind at the airplane’s parked location, which led to turbulent gust loads on the right elevator sufficient to jam it, even though the horizontal surface wind speed was below the certification design limit and maintenance inspection criteria for the airplane, and (2) the lack of a means to enable the flight crew to detect a jammed elevator during preflight checks for the Boeing MD-83 airplane. Contributing to the survivability of the accident was the captain’s timely and appropriate decision to reject the takeoff, the check airman’s disciplined adherence to standard operating procedures after the captain called for the rejected takeoff, and the dimensionally compliant runway safety area where the overrun occurred.
Source: NTSB AAR 19/01, ¶3.2
References
(Source material)
Boeing Flight Operations Bulletin, MD-80-01-02, Flight Control Jam, June 25, 2001
NTSB Aircraft Accident Report, AAR-19/01, Runway Overrun During Rejected Takeoff, Ameristar Air Cargo, Inc., dba Ameristar Charters, flight 9363, Boeing MD-83, N786TW, Ypsilanti, Michigan, March 8, 2017
NTSB Group Chairman's Factual Report, DCA17/FA076, September 6, 2017