The cause of this mishap, that is the thing that started it all, were tires that were found to be 36 percent under-inflated. Learjet procedure called for checking the tires before the first flight of each day or every 10 days they are not in use, but they hadn't been checked for three weeks. The Learjet maintenance manual further stipulates that a tire should be replaced if it ever falls below 15 percent. The organization's director of maintenance was unaware of this requirement and it appears the tires on the accident airplane had not be checked in three weeks. An underinflated tire is prone to fail and that's what happened here.
— James Albright
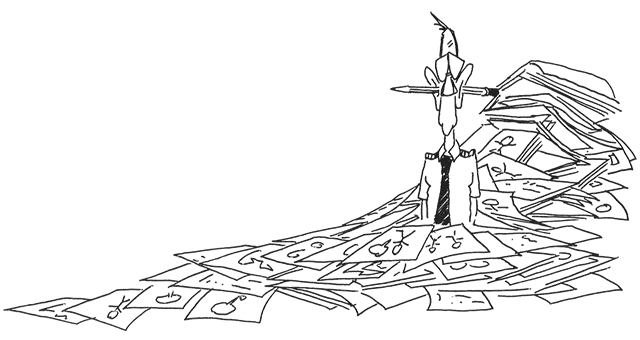
Updated:
2018-02-26
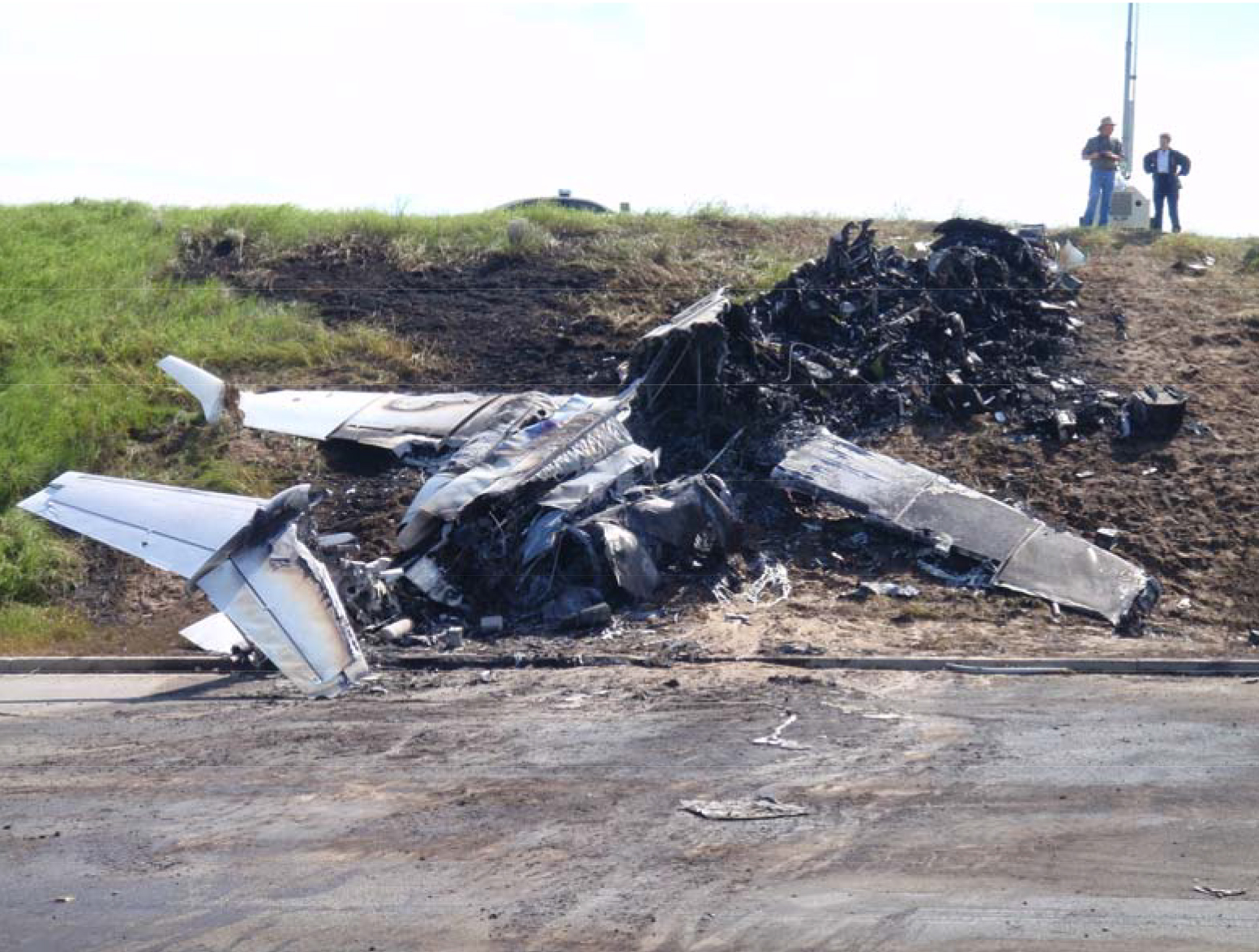
Airplane Wreckage,
NTSB Accident Docket, DCA08MA098, Photo 1
There are more lessons here than just proper tire inflation. The thrust reversers didn't behave as expected due to damage of other systems by the tire. Despite all that, the accident was survivable had proper abort procedures been used. But is all started with the tires.
So let's assume your tires are checked before the first flight of the day whenever you depart home plate. But what about on the road? The NTSB report brought up some interesting points on who can check tires, legally, and who cannot:
- In correspondence dated January 8, 2009, Learjet requested that the FAA provide a legal interpretation of “applicable rules in 14 CFR Parts 43, 91, and 135 pertaining to whether a pilot of a transport-category aircraft may check tire pressure during a normal preflight inspection.” On February 26, 2009, the FAA’s assistant chief counsel for regulations responded that checking the tire pressure on a Learjet 60 airplane is preventive maintenance and not a simple preflight task. The FAA stated that such checks involve high air pressure and require a calibrated gauge that must be used properly to ensure correct readings.
- Title 14 CFR 43.3(g) allows pilots to perform preventive maintenance on an aircraft operating under Part 91 but not aircraft operated under Parts 121, 129, or 135. The FAA noted that, “accordingly, a pilot operating [a Learjet 60] under … Part 91 may, in accordance with the provisions of 14 CFR 43.3(g), perform daily landing gear tire pressure checks. Under the same regulation, however, a pilot of that aircraft operating under Part 135 may not perform that task.” The FAA stated that any Part 135 Learjet 60 operator that may be adversely affected by the maintenance requirement may petition the FAA for relief from the regulation.
Source: NTSB AAR-10/02, ¶1.18.2.4
I think you should have a firm understanding of the tire pressure loss pattern of your aircraft and measure the pressure when on the road at appropriate intervals. If you are flying under 14 CFR 91, you should have your mechanic train you for the task and equip the aircraft with a properly calibrated gauge. If you are flying under 14 CFR 135, you need to make the appropriate arrangements to have a qualified mechanic perform the task.
Maintenance teams do not always face the same scrutiny as pilots. The FAA performed 159 inspections of one sort or another on this 14 CFR 135 organization but never detected what had to have been a lax attitude towards regular tire pressure checks. Smaller operations, especially those with a single mechanic, are susceptible to the pressure to take short cuts, may lack a quality assurance structure, and can suffer from poor training. These, with the added factor of fatigue, make up what I call: Maintenance Malpractice
Now onto the second problem: takeoff abort procedures. This pilot initiated the abort above V1, thought better of it, and then decided to abort again. The initial abort began two seconds after the first officer called V1. I think had the captain remained committed to the abort, even though it was after V1, they could have survived the stop. But as the takeoff progressed the main landing gear weight on wheels switches were damaged and the thrust reversers stowed and her throttle inputs resulted in forward thrust. They departed the runway well over 100 knots. More about decision speed here: V1.
Why would a properly trained pilot have such a problem with an abort decision after V1? The NTSB report hypothesizes she may have been startled by the unexpected tire failure, which in the cockpit was a loud noise, the right wing dropping a few inches, and vibration. Back in the days when we expected a tire failure now and then you got used to it. These days we need to practice the scenario in a simulator. I think if you have experience with the world falling apart around you, this might not be an issue for you. But if your aviation experience has been nothing but blue skies from the day you started flying, you should make an effort to load yourself up in a simulator.
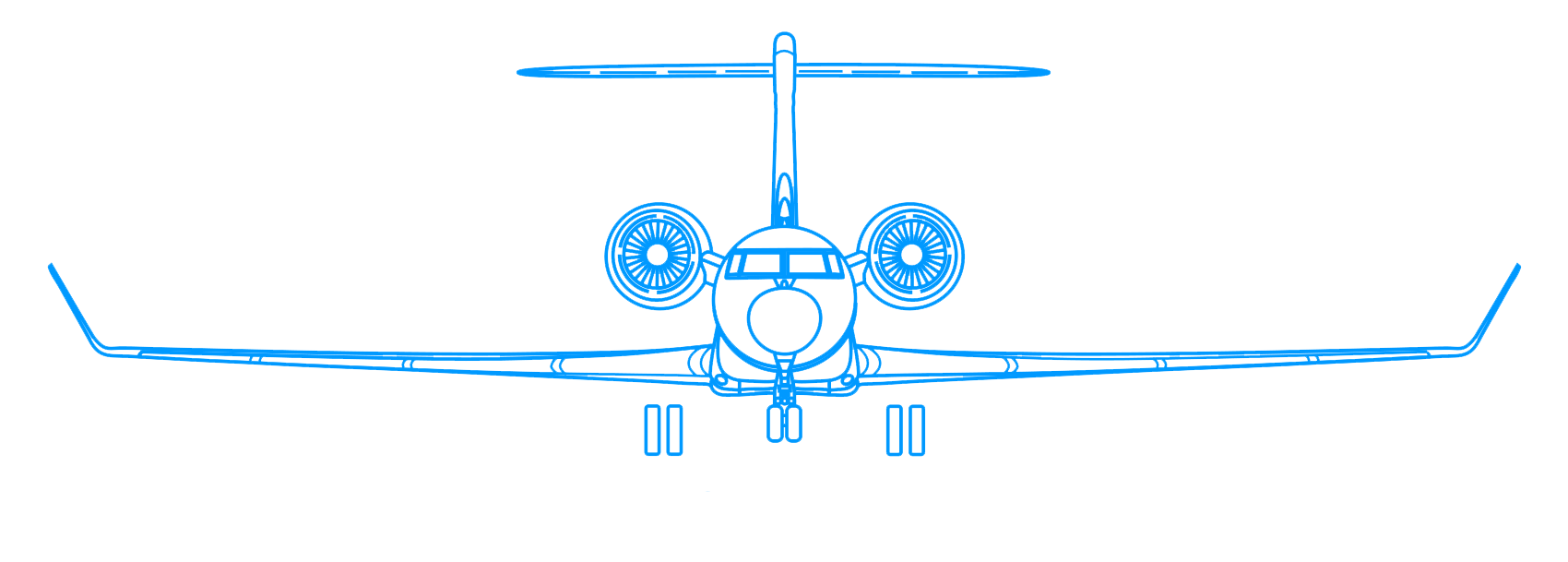
1
Accident report
- Date: 19 SEP 2008
- Time: 23:53
- Type: Learjet 60
- Operator: Global Exec Aviation
- Registration: N999LJ
- Fatalities: 2 of 2 crew, 4 of 6 passengers
- Aircraft Fate: Destroyed
- Phase: Takeoff
- Airport: (Departure) Columbia Metropolitan Airport, SC (CAE/KCAE), United States of America
- Airport: (Destination) Los Angeles-Van Nuys Airport, CA (VNY), United States of America
2
Narrative
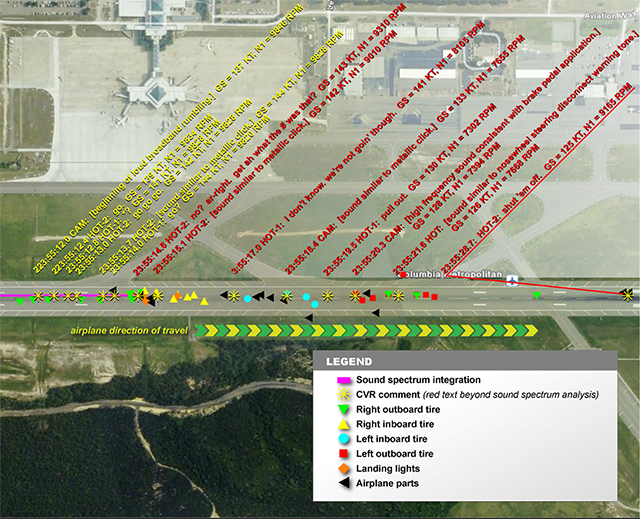
Map of Columbia Metropolitan Airport, showing integrated sound spectrum data, CVR comments, and wreckage locations plotted, from NTSB AAR-10/02, figure 6.
At 2351:22, the captain briefed the RTO procedure and stated, “we’ve got plenty of runway so we’ll abort for anything below eighty knots after V-one and before V-two engine failure fire malfunction loss of directional control all the big things after V-two we’ll go ahead and take it into the air treat it as an in-flight emergency.” The first officer replied, “correct.” The captain asked if the first officer had any questions, and the first officer asked, “reference the ah between eighty and ah V-one you’re only ah aborting for the fire failure loss of directional control?” The captain replied, “yes,” then added, “or an inadvertent thrust-, ah, T-R [thrust reverser] deployment.” The first officer then stated, “that will ah cause the loss of directional control I guess,” to which the captain replied, “exactly hah they go together.” The first officer later stated, “well eh if the runway is long I abort but if it’s short I kinda do different briefing depending on what the length of the runway is but we’re pretty heavy so it’s probably not a bad idea.”
Source: NTSB AAR-10/02, ¶1.1
The captain's briefing is not only contrary to her company's procedures, it is wrong. V1 by definition is the speed at which the abort decision has been made, not V2.
The captain initiated the takeoff roll, and, at 2355:00.1, the first officer stated, “eighty knots. Crosscheck,” to which the captain replied, “check.” At 2355:10.5, the first officer reported, “V-one.” About 1.5 seconds later, the CVR captured the beginning of a loud rumbling sound. Postaccident sound spectrum and airplane performance studies indicated that the airplane’s position on the runway at the onset of the loud rumbling sound corresponded with the location where the first main landing gear (MLG) tire fragments were found. Four-tenths second after the beginning of the loud rumbling sound, the first officer stated, “go,” the captain stated something unintelligible, and, at 2355:13.0, the first officer stated, “go go go.” The CVR recorded a sound similar to a metallic click, and, at 2355:14.0, the captain stated, “go?” Postaccident sound spectrum and airplane performance studies estimated that, about this time, the airplane’s ground speed reached a peak of about 144 kts. The first officer then stated, “no? ar- alright. Get ah what the [expletive] was that?” The CVR recorded another metallic click sound, and, at 2355:17.0, the captain stated, “I don’t know. We’re not goin’ though.”
At 2355:18.4, another metallic click sound was recorded, and, at 2355:19.5, the captain stated, “full out.” Postaccident performance studies indicated that the airplane was decelerating. Within 1 second, the CVR captured a sound consistent with the application of wheel braking, and, at 2355:21.6, the CVR captured a sound consistent with the nosewheel steering disconnect warning tone. Postaccident performance studies indicated that the airplane had then accelerated. About 7 seconds later, the first officer stated, “shut ’em off,” and, at 2355:32.4, the first officer stated, “they’re shut off they’re shut off.” At 2355:36.0, the first officer made a radio transmission on the CAE tower control frequency, saying, “roll the equipment we’re goin’ off the end.” The CVR recording ended at 2355:41.1.
Source: NTSB AAR-10/02, ¶1.1
The captain, age 31, held an airline transport pilot (ATP) certificate for multiengine land airplanes with type ratings for Cessna CE-500 (issued on June 18, 2005), Learjet 60 (issued on October 25, 2007), and Cessna CE-650 (issued on January 19, 2008) airplanes.
A review of FAA records found no previous accidents, incidents, or enforcement actions. FAA records indicated that the captain received a notice of disapproval on August 11, 2006, for a practical test for the ATP certificate because of unsatisfactory performance in the non-precision approach and circle-to-land procedures. She retested the same day and passed. On April 11, 1997, when the captain was a private pilot with about 192 total flight hours, she received a notice of disapproval for the practical test for the airplane instrument rating because of unsatisfactory performance of partial-panel very high frequency omnidirectional radio range (VOR) instrument approach procedures and instrument landing system instrument approach procedures. She was retested on April 14, 1997, and received a second notice of disapproval because of unsatisfactory performance of partial-panel VOR instrument approach procedures. She was retested on May 28, 1997, and passed. On November 14, 1997, when the captain had accrued about 252 total flight hours, she received a notice of disapproval for a practical test for the commercial airplane multiengine land certificate because of unsatisfactory knowledge of the national airspace system and airplane performance and limitations. (She subsequently passed the checkride for private pilot privileges for multiengine land airplanes on December 6, 1997, and she passed the checkride for the commercial certificate for multiengine land airplanes on September 1, 2004.12)
The first officer, age 52, held an ATP certificate for multiengine land airplanes with type ratings for Learjet 60 (issued on March 1, 2007) and Cessna CE-500 airplanes. He held a first-class airman medical certificate issued July 18, 2008, with the limitations “must wear corrective lenses” and “possess glasses for near/intermediate vision.” According to employment records from Global Exec Aviation and estimates from another employer and a previous employer, the first officer had accumulated about 8,200 hours total flight time, including about 7,500 hours PIC time and about 300 hours in Learjet 60 airplanes (about 108 hours of which were as SIC). In the 90 days, 30 days, and 24 hours preceding the accident, the first officer logged about 42, 34, and 1.5 hours, respectively.
Source: NTSB AAR-10/02, ¶1.5
The airplane was equipped with dual wheel and tire assemblies at each MLG position. Each MLG tire was a Goodyear Flight Eagle, part number (P/N) 178K43-1, size 17.5 x 5.75-8.18 For use on the Learjet 60, the rated tire inflation pressure was 220 pounds per square inch (psi). Applicable tire certification requirements are specified in 14 CFR 25.733 and FAA Technical Standard Order (TSO) TSO-C62c. TSO-C62c specified various tire performance criteria, one of which was a maximum allowable air pressure loss of 5 percent per day for an airplane tire under normal operating circumstances. According to the Goodyear Qualification Test Report (QTR) 461B-3044-TL, the Goodyear Flight Eagle tire documented a daily pressure loss of about 2.2 percent.
Maintenance logs indicated that all four MLG tires were new when installed in December 2007 and had accumulated a total of 20 landings at the time of the accident. Flight history records showed that the airplane had flown 5 days during the 12-day period that preceded the accident. Interviews with personnel from all facilities that handled the accident airplane during that time period revealed that none had serviced or received a request to service the MLG tires. Global Exec Aviation’s director of maintenance estimated that the tire pressures may not have been checked for about 3 weeks before the accident.
The contents of chapter 12 of the AMM [Learjet 60 Aircraft Maintenance Manual] related to technical specifications and descriptions of how to perform various maintenance tasks. Chapter 12-10-05, pages 301 and 302, contained the following guidance:
Important inflation practices and tips are as follows: ... Measure the cold tire pressure before the first flight of every day or every 10 day[s] on in-service tires [that] are not in use.... Do not underinflate the tire. An underinflated tire generally cannot be detected visually.
The AMM indicated that a tire should be replaced if found to have operated at an inflation pressure loss of 15 percent.
Source: NTSB AAR-10/02, ¶1.6
3
Analysis
The Captain
Interviews with other pilots, a Learjet 60 proficiency check evaluator, and flight and ground training instructors who were familiar with the captain’s flying and training in recent years revealed that none expressed any concerns about the captain’s competence.
Source: NTSB AAR-10/02, ¶2.1
The captain’s action to reject the takeoff and her lack of a callout, contrary to her training, may have been the result of the “startle factor,” which is often lacking in training scenarios. In most V1 training scenarios, pilots are in a simulator, are aware that they will be receiving an anomaly (usually an engine failure) on takeoff, and are prepared to respond. In the real world, the situation is more dynamic, the consequences are greater, and the pilot is not aware that a failure will occur or what type of failure it is. This “startle factor” can increase the stress level of the pilot, resulting in an incorrect decision being made.
Source: NTSB AAR-10/02, ¶2.4
Tires
Transport-category aircraft tires, such as those installed on the Learjet 60, operate at high inflation pressures and have a thick-walled construction made up of three main materials: rubber, fabric (primarily the flexible nylon ply material that gives tires their strength), and the steel bead wires.
Pieces of the right outboard tire, which were the first debris found on the runway, were identified as fragments of the tire’s sidewall. About 80 percent of the tire was reconstructed. Both sidewalls showed damage around the entire circumference of the tire; the damage had a ragged appearance and was located at a generally uniform distance from the wheel rim.
Both the inboard right tire and the inboard left tire showed similar sidewall damage (on one sidewall each). The outboard left tire, fragments of which were the farthest down the runway, had a more torn and shredded appearance than the other three tires and showed extensive tearing through its layers. One fragment of this tire, about 19 inches in circumferential length, showed sidewall damage.
The characteristics of the sidewall damage observed on all four of the accident airplane’s tires were consistent with a photograph in a Goodyear publication showing typical heat damage sustained from sidewall overdeflection and flexing fatigue. Goodyear engineers and the Goodyear publication noted that previous tire testing found that aircraft tire sidewall damage from flexing fatigue is predominantly consistent with taxi-cycle operations while the tire is underinflated; Goodyear testing showed that as little as 5-percent underinflation greatly reduces the fatigue life of transport-category aircraft tires.
The tires showed no evidence of impact, puncture, or adhesive-separation damage. Examination of the wheels and brakes showed no evidence of overheating or brake lock-up, and none of the wheels’ eutectic fuse plugs, which are designed to melt if the wheel temperature reaches about 390° F, leaked when tested.
Source: NTSB AAR-10/02, ¶1.16.3
The relatively new set of tires on the accident airplane showed no evidence of failures in design, manufacturing flaws, or exterior damage, such as punctures or other damage from striking foreign objects. Therefore, the NTSB concludes that the following were not factors in this accident: tire design, tire manufacture, or damage to the exterior of any tire.
Source: NTSB AAR-10/02, ¶2.1
Damage observed on fragments from all four MLG tires, such as abrasion marks on the inner liner and heat damage to the rubber and nylon materials, was consistent with overdeflection of the tires. Two tire operating conditions can result in such sidewall overdeflection: overloading and underinflation. Under either condition, excessive flexing of the sidewall generates high internal temperatures and weakening of the sidewall plies, leading directly to failure.
The location of the sidewall damage on each of the accident airplane’s tires was helpful in determining which condition resulted in or contributed to the tire failures. Static test data show that, if proper inflation of all four MLG tires is assumed, to achieve the amount of overdeflection evident from the damage, each tire would need to be overloaded with about 12,200 pounds (about twice as much weight as the accident airplane could have imposed on each tire). Such overloading of four properly inflated tires is implausible (it would require an airplane gross weight in excess of 48,800 pounds), and other evidence strongly supports that the tires were not properly inflated.
Global Exec Aviation’s director of maintenance indicated that he did not know how often the Learjet 60’s tire pressures should be checked but that he referred to the respective AMM for each type of airplane operated by the company to know when to perform scheduled maintenance items.
Allowing the airplane to operate for weeks without a tire pressure check is inconsistent with all available guidance.
Other inconsistencies related to tire pressure checks were found in the FAA’s February 26, 2009, response to Learjet regarding the Learjet 60. In the letter, the FAA stated that checking the tires on a Learjet 60 is preventive maintenance, which pilots would not be permitted to do as part of a preflight check. However, the FAA further explained that a pilot flying a Learjet 60 under 14 CFR Part 91 may perform tire pressure checks but that a pilot flying a Learjet 60 under 14 CFR Part 135 may not.
Source: NTSB AAR-10/02, ¶2.3.1
Thrust Reversers
The Learjet 60 is equipped with cockpit annunciator lights that indicate the status of the thrust reverser system to the pilots. Ground tests were performed using a Learjet 60 that was specially equipped to simulate possible anomalies that could affect the thrust reverser system’s logic functions, and the test airplane’s cockpit annunciator lights were monitored throughout the testing.
Source: NTSB AAR-10/02, ¶1.16.4
Going heads down for a look at thrust reverser annunciators is extraordinarily hard to do, and I would say inadvisable. (Your eyes need to be outside.) It is a good practice to have the nonflying pilot announce thrust reverser status.
During one test, the airplane was on the ground (with squat switches in ground mode) with the thrust reversers deployed and at idle thrust. While the airplane remained on the ground, the test equipment switched the squat switch status to air mode, thus creating a situation in which the logic requirements to maintain thrust reverser deployment were no longer being met. As a result, the thrust reverser doors stowed, the TR DEPLOY light extinguished, the TR UNLOCK light illuminated, and the TR ARM light flashed for less than 2 seconds before all thrust reverser system annunciators extinguished. The EECs, upon receiving the input that the thrust reversers were stowed and that the squat switches were signaling air mode, shifted logic and signaled the FADEC components to change the engine thrust output from the reverse thrust power schedule to the forward thrust power schedule. (In the test airplane’s configuration, the thrust changed from ground idle speed to flight idle speed.)
Source: NTSB AAR-10/02, ¶1.16.4
Some aircraft will command idle thrust at this point or give an audible warning.
In this situation, the EECs would transition from the reverse thrust power schedule to the forward thrust power schedule during about a 2-second transition through idle power. During the entire sequence, the thrust reverser levers in the cockpit would remain in the reverse thrust idle position (as selected by the pilot) while the engines produced forward thrust. Because both the thrust reverser levers and the forward thrust levers share common RVDTs (one for the left engine and one for the right engine), the EECs, which receive TLA information from the RVDTs, would signal the engines to produce a level of forward thrust that generally corresponds with the level of reverse thrust commanded; that is, a pilot commanding full reverse thrust (for maximum deceleration of the airplane) would instead receive high levels of forward thrust (accelerating the airplane) according to the forward thrust power schedule. To reduce the forward thrust in such a situation in a Learjet 60, a pilot would need to move the thrust reverser levers out of the commanded reverse thrust position and place them in the stowed position, consistent with the “Inadvertent Stow of Thrust Reverser After a Crew-Commanded Deployment” procedure.
Source: NTSB AAR-10/02, ¶1.16.4
This is obviously a poor design and probably an unexpected behavior.
The fail-safe concept for the Learjet 60 thrust reverser system design was intended to protect against deployment of thrust reversers in flight. To achieve this protection, the logic criteria were such that any system failures or anomalies would result in the stowage of the thrust reversers. An uncommanded stowage of the thrust reversers in the Learjet 60 does not result in a corresponding movement of the cockpit thrust reverser levers.
Source: NTSB AAR-10/02, ¶1.16.4
Rejected Takeoff
During the takeoff, when the first tire failed and the rumbling noise began, the first officer stated, “go,” then “go, go, go.” The airplane’s ground speed at the time was about 137 kts, and, as shown by runway gouging and tire skid marks, the airplane veered to the right and across the runway centerline. Only debris from the right outboard tire was found at the runway location that coincided with the timing of this event; thus, the runway marks were likely created by the right outboard wheel rim contacting the runway surface and the skidding of the still-intact right inboard tire. (The airplane was initially left of the runway centerline before it veered.) Hydraulic fluid, consistent with that found in at least one severed brake hose, was present on some tire fragments.
Source: NTSB AAR-10/02, ¶2.2
At this point an abort could probably have been made successfully, with just one failed tire and enough runway remaining. A continued takeoff, however, would allow the airplane to attempt a landing at a lower weight, a lower speed, and much more runway ahead.
In the next second (2 seconds after the onset of the rumbling sound), the captain asked, “go?” At this point in the takeoff roll, the airplane neared its peak ground speed of about 144 kts (extrapolated data show that it may have reached about 150 kts within the next second) and began shedding fragments of a landing light and other pieces (which likely separated after having been impacted by fragments of the right outboard tire). The timing of the captain’s question to the first officer coincided with the captain reducing engine power for about 1 second, then increasing it for about 1 second before decreasing it again, about which time the first officer stated, “no? ar-right ... what the [expletive] was that?” The entire RTO procedure, up to this point, spanned about 5 seconds since the onset of the rumbling noise from tire fragments, first from the right outboard tire and then from the right inboard tire.
Source: NTSB AAR-10/02, ¶2.2
The first officer's "go, go, go" callout was a good one but it needed to be followed up after the captain asked "go?" The first officer could have also been more forceful during the captain's botched takeoff briefing on the very subject of rejecting the takeoff.
Thus, the NTSB considered the possibility that the captain rejected the takeoff because of a perceived loss of airplane control. However, runway markings show that, after the airplane veered, it was realigned with the runway heading, indicating that the captain was able to regain and maintain directional control. Further, although the captain initially reduced engine power, she did not make an RTO callout, and her subsequent increase in engine power, concurrent with her question “go?,” indicates that she briefly considered continuing the takeoff before finally committing to the RTO. Therefore, the NTSB concludes that the captain’s uncertainty as to whether to continue the takeoff suggests that her initial action to reject it did not result from a perception that the airplane was uncontrollable and could not fly.
As supported by the airplane performance study discussed in section 1.16.2, after the captain had committed to performing the high-speed RTO, the accident airplane’s thrust reverser system initially performed as commanded. On the basis of the captain’s comment “full out,” which coincided with a noticeable deceleration of the airplane, the study found that the thrust reversers had fully deployed, and the system provided reverse engine thrust. However, about 7 seconds after the captain committed (about 10 seconds after the rumbling noise began), the CAM [Cockpit Area Microphone] captured the nosewheel steering disconnect warning tone. Because nosewheel steering is typically engaged while the airplane is on the ground, the timing of this tone provides an indication of when the system status changed to “air mode.” For this to occur, the circuit associated with the MLG electrical components and wiring, which include the wheel speed sensor and squat switch, must have sustained damage that affected the air-ground signal. Debris found on the runway and other physical evidence show that the MLG area where system components were mounted sustained damage from the shedding tire fragments.
As a result, because the system logic requirements for maintaining thrust reverser deployment were no longer being met, the thrust reversers stowed. Meanwhile, as indicated by engineering and ground tests, the thrust reverser levers in the cockpit remained in the raised full-reverse-thrust position, the TR DEPLOY annunciators extinguished, and the TR UNLOCK annunciators momentarily illuminated (while the reverser doors were in transit toward stow); then, the TR ARM annunciators flashed briefly before all TR annunciators extinguished completely. During this sequence, the EECs shifted logic and signaled the FADEC components to change to the forward thrust power schedule. Because the flight crew had commanded full reverse thrust, the EECs (because of the shifted logic) interpreted the TLA and RDVT positions and signaled the FADEC components to provide forward thrust at near takeoff power.
As a result, the flight crew was faced with an emergency in which the wheel braking system was compromised due to tire and hydraulic system damage, and the airplane was accelerating with high engine power toward obstacles beyond the end of the runway. There was no warning annunciator in the cockpit to indicate any system anomaly; thus, initially, the captain was expecting to have continued reverse thrust as commanded with the reverse thrust levers. Under these abnormal circumstances, reducing the airplane’s uncommanded forward thrust would require moving the thrust reverser levers to the stowed position. However, moving the reverser levers to the stowed position (when maximum reverse thrust was needed and commanded) during an RTO was likely counterintuitive to the captain.
Source: NTSB AAR-10/02, ¶2.2
4
Cause
The National Transportation Safety Board determines that the probable cause of this accident was the operator’s inadequate maintenance of the airplane’s tires, which resulted in multiple tire failures during takeoff roll due to severe underinflation, and the captain’s execution of a rejected takeoff after V1, which was inconsistent with her training and standard operating procedures.
Contributing to the accident were (1) deficiencies in Learjet’s design of and the Federal Aviation Administration’s (FAA) certification of the Learjet Model 60’s thrust reverser system, which permitted the failure of critical systems in the wheel well area to result in uncommanded forward thrust that increased the severity of the accident; (2) the inadequacy of Learjet’s safety analysis and the FAA’s review of it, which failed to detect and correct the thrust reverser and wheel well design deficiencies after a 2001 uncommanded forward thrust accident; (3) inadequate industry training standards for flight crews in tire failure scenarios; and (4) the flight crew’s poor crew resource management.
Source: NTSB AAR-10/02, ¶2.2
References
(Source material)
NTSB Aircraft Accident Report, AAR-10/02, Runway Overrun During Rejected Takeoff, Global Exec Aviation, Bombardier Learjet 60, N999LJ, Columbia, South Carolina, September 19, 2008