Thirty years ago spatial disorientation was a leading cause of military aircraft accidents and it may still be. In the civilian world of transporting people and things from Point A to Point B, it takes a much smaller toll because very few of us intentionally pull more than a G and a half or ever venture beyond a standard rate turn. But in the world of instrument flight, it is a threat.
— James Albright
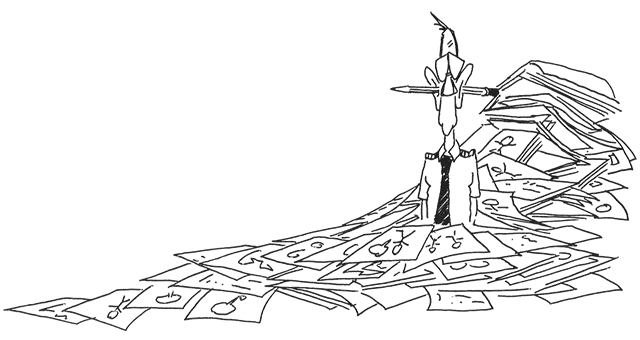
Updated:
2018-01-20
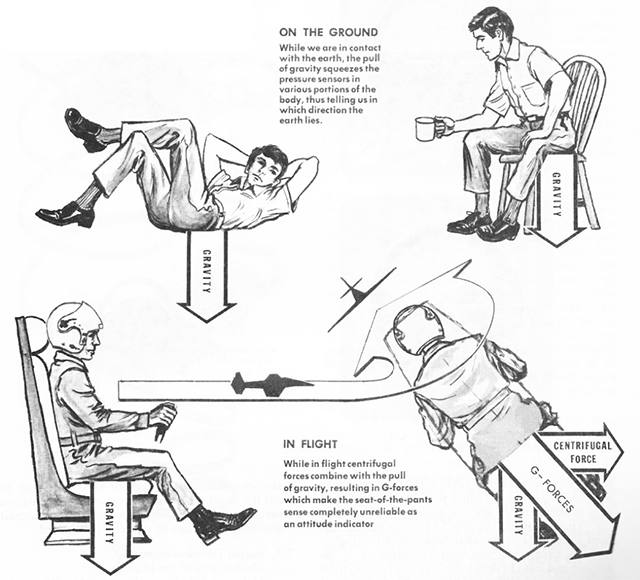
Somatosensory System,
from AFM 51-37, Figure 7-3.
The cure, for an instrument pilot, is to stay on instruments. But sometimes that is easier said than done. The cure, in that case, is knowledge. If you know about what can happen, you will be better prepared to deal with it when it does.
I was in the right seat of an Air Force Boeing 707, while one of the sharpest pilots I've ever known was in the left seat. We were both instructor pilots and the night was for proficiency in receiver air refueling. It was a moonless night over the ocean with no external references other than the lights on the tanker. He was doing a great job until the first turn. "Take the airplane," he said. "Take it now." I took the aircraft and he moved his head from the tanker to his attitude indicator. He said he got the leans all of a sudden. He never experienced them before, but he knew what the problem was before it happened.
1 — Common terms of spatial disorientation
2 — Types of spatial disorientation
4 — Illusions leading to spatial disorientation
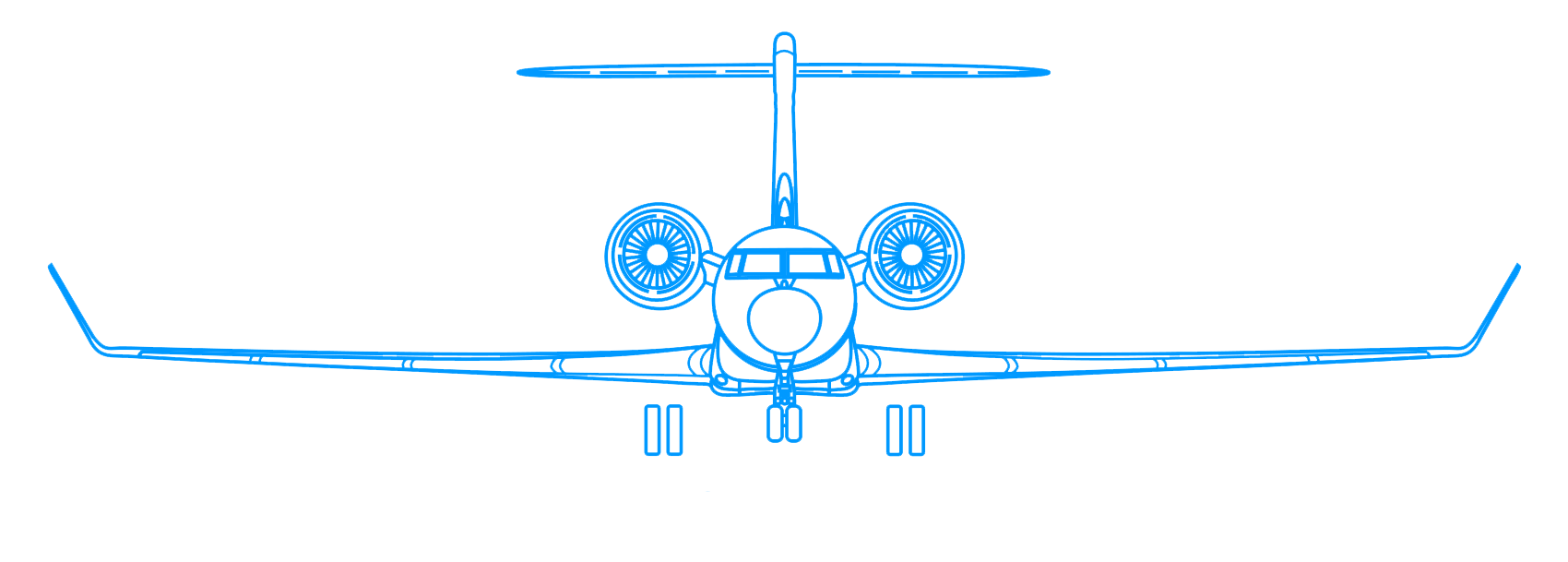
1
Common terms of spatial disorientation
Spatial disorientation
Spatial disorientation is an individual’s inability to determine his or her position, attitude, and motion relative to the surface of the earth or significant objects; for example, trees, poles, or buildings during hover. When it occurs, pilots are unable to see, believe, interpret, or prove the information derived from their flight instruments. Instead, they rely on the false information that their senses provide.
Source: U.S. Army Aeromedical Training Manual, ¶9-1.
Spatial disorientation is not unusual, a properly trained pilot may have a moment of disorientation but "pulls out of it" once recognized.
Sensory Illusion
A sensory illusion is a false perception of reality caused by the conflict of orientation information from one or more mechanisms of equilibrium. Sensory illusions are a major cause of spatial disorientation.
Source: U.S. Army Aeromedical Training Manual, ¶9-2.
We used to get this all the time while air refueling. Our eyes were on the wings of the tanker, which became our local horizon. A cloud deck below or the actual earth's horizon could disagree with the tanker's attitude and we could often find ourselves with momentary disorientation. Every now and then it got so bad we had to exchange aircraft control between pilots.
You can get it in every day flight by things like a sloping cloud deck or even a long, steady turn. The key, of course, is to check your attitude and performance instruments.
Vertigo
Vertigo is a spinning sensation usually caused by a peripheral vestibular abnormality in the middle ear. Aircrew members often misuse the term vertigo, applying it generically to all forms of spatial disorientation or dizziness.
Source: U.S. Army Aeromedical Training Manual, ¶9-3.
2
Types of spatial disorientation
This Army manual seems to assume there is a copilot present to gain control of the aircraft. There have been cases where the copilot was unwilling to assume command for fear of angering the pilot, such as: Korean Air 8509 or where the rest of the crew was probably not engaged actively in backing up the captain because the culture of their airline was that the captain could never be challenged, as in: Pan American World Airways 816.
Type I (Unrecognized)
A disoriented aviator does not perceive any indication of spatial disorientation. In other words, he does not think anything is wrong. What he sees—or thinks he sees—is corroborated by his other senses. Type I disorientation is the most dangerous type of disorientation. The pilot—unaware of a problem—fails to recognize or correct the disorientation, usually resulting in a fatal aircraft mishap:
- The pilot may see the instruments functioning properly. There is no suspicion of an instrument malfunction.
- There may be no indication of aircraft-control malfunction. The aircraft is performing normally.
- An example of this type of SD would be the height-/depth-perception illusion when the pilot descends into the ground or some obstacle above the ground because of a lack of situational awareness.
Source: U.S. Army Aeromedical Training Manual, ¶9-4.
Type II (Recognized)
In Type II spatial disorientation, the pilot perceives a problem (resulting from spatial disorientation). The pilot, however, may fail to recognize it as spatial disorientation:
- The pilot may feel that a control is malfunctioning.
- The pilot may perceive an instrument failure as in the graveyard spiral, a classic example of Type II disorientation. The pilot does not correct the aircraft roll, as indicated by the attitude indicator, because his vestibular indications of straight and level flight are so strong.
Source: U.S. Army Aeromedical Training Manual, ¶9-5.
Type III (Incapacitating)
In Type III spatial disorientation, the pilot experiences such an overwhelming sensation of movement that he or she cannot orient himself or herself by using visual cues or the aircraft instruments. Type III spatial disorientation is not fatal if the copilot can gain control of the aircraft.
Source: U.S. Army Aeromedical Training Manual, ¶9-6.
3
Equilibrium maintenance
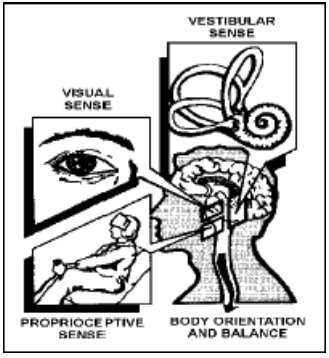
The Three Equilibrium Systems, from U.S. Army Aeromedical Training Manual, Figure 9-1.
The important lesson here is we tend to believe our vestibular and proprioceptive systems naturally, but it is our visual system that is most reliable. We have to train ourselves to overrule the vestibular and proprioceptive systems in favor of the visual.
Three sensory systems—the visual, vestibular, and proprioceptive systems—are especially important in maintaining equilibrium and balance. Figure 9-1 shows these systems. Normally, the combined functioning of these senses maintains equilibrium and prevents spatial disorientation. During flight, the visual system is the most reliable. In the absence of the visual system, the vestibular and proprioceptive systems are unreliable in flight.
Source: U.S. Army Aeromedical Training Manual, ¶9-7.
Visual System
Of the three sensory systems, the visual system is the most important in maintaining equilibrium and orientation. To some extent, the eyes can help determine the speed and direction of flight by comparing the position of the aircraft relative to some fixed point of reference. Eighty percent of our orientation information comes from the visual system.
Source: U.S. Army Aeromedical Training Manual, ¶9-8.
Vestibular System
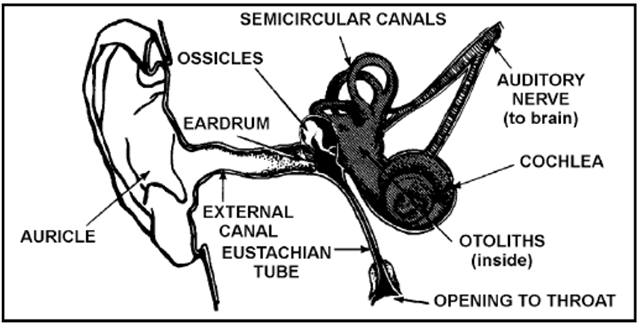
The Vestibular System, from U.S. Army Aeromedical Training Manual, Figure 9-2.
The inner ear contains the vestibular system, which contains the motion- and gravity detecting sense organs. This system is located in the temporal bone on each side of the head. Each vestibular apparatus consists of two distinct structures: the semicircular canals and the vestibule proper, which contain the otolith organs. [The figure] depicts the vestibular system. Both the semicircular canals and the otolith organs sense changes in aircraft attitude. The semicircular canals of the inner ear sense changes in angular acceleration and deceleration.
Source: U.S. Army Aeromedical Training Manual, ¶9-11.
Otolith Organs
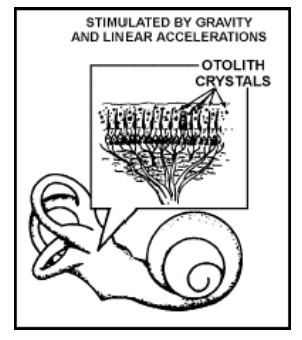
The Otolith Organs, from U.S. Army Aeromedical Training Manual, Figure 9-3.
The otolith organs are small sacs located in the vestibule. Sensory hairs project from each macula into the otolithic membrane, an overlaying gelatinous membrane that contains chalk-like crystals, called otoliths. The otolith organs, shown in [the figure], respond to gravity and linear accelerations/decelerations. Changes in the position of the head, relative to the gravitational force, cause the otolithic membrane to shift position on the macula. The sensory hairs bend, signaling a change in the head position.
Source: U.S. Army Aeromedical Training Manual, ¶9-12.
When the head is upright, a "resting" frequency of nerve impulses is generated by the hair cells.
Source: U.S. Army Aeromedical Training Manual, ¶9-13
When the head is tilted, the "resting" frequency is altered. The brain is informed of the new position.
Source: U.S. Army Aeromedical Training Manual, ¶9-14.
Linear accelerations/decelerations also stimulate the otolith organs. The body cannot physically distinguish between the inertial forces resulting from linear accelerations and the force of gravity. A forward acceleration results in backward displacement of the otolithic membranes. When an adequate visual reference is not available, aircrew members may experience an illusion of backward tilt.
Source: U.S. Army Aeromedical Training Manual, ¶9-15.
Semicircular Canals
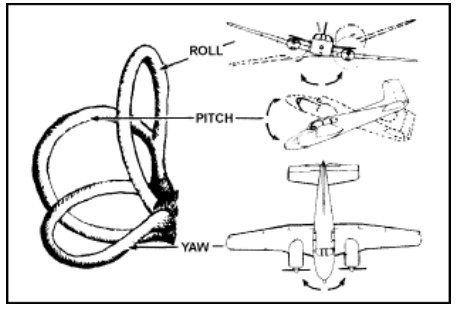
Reaction of the Semicircular Canals to Changes in Angular Acceleration, from U.S. Army Aeromedical Training Manual, Figure 9-7.
The semicircular canals of the inner ear sense changes in angular acceleration. The canals will react to any changes in roll, pitch, or yaw attitude.
Source: U.S. Army Aeromedical Training Manual, ¶9-16.
The semicircular canals are situated in three planes, perpendicular to each other. They are filled with a fluid called endolymph. The inertial torque resulting from angular acceleration in the plane of the canal puts this fluid into motion. The motion of the fluid bends the cupula, a gelatinous structure located in the ampulla of the canal. This, in turn, moves the hairs of the hair cells situated beneath the cupula. This movement stimulates the vestibular nerve. These nerve impulses are then transmitted to the brain, where they are interpreted as rotation of the head.
Source: U.S. Army Aeromedical Training Manual, ¶9-17.
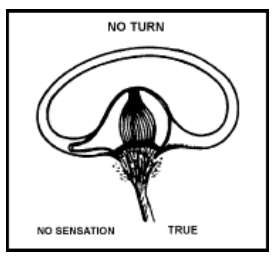
Position of Hair Cells During No Acceleration, from U.S. Army Aeromedical Training Manual, Figure 9-9.
When no acceleration takes place, the hair cells are upright. The body senses that no turn has occurred.
Source: U.S. Army Aeromedical Training Manual, ¶9-18.
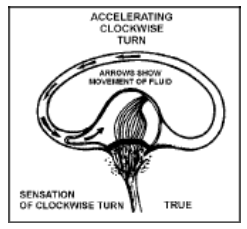
Sensation During a Clockwise Turn, from U.S. Army Aeromedical Training Manual, Figure 9-10.
When a semicircular canal is put into motion during clockwise acceleration, the fluid within the semicircular canal lags behind the accelerated canal walls. This lag creates a relative counterclockwise movement of the fluid within the canal. The canal wall and the cupula move in the opposite direction from the motion of the fluid. The brain interprets the movement of the hairs to be a turn in the same direction as the canal wall. The body correctly senses that a clockwise turn is being made.
Source: U.S. Army Aeromedical Training Manual, ¶9-19.
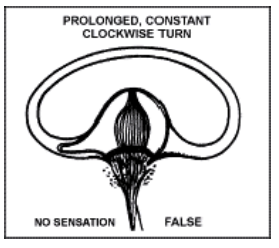
Sensation During a Prolonged Clockwise Turn, from U.S. Army Aeromedical Training Manual, Figure 9-11.
If the clockwise turn then continues at a constant rate for several seconds or longer, the motion of the fluid in the canals catches up with the canal walls. The hairs are no longer bent, and the brain receives the false impression that turning has stopped. The position of the hair cells and the resulting false sensation during a prolonged, constant clockwise turn is shown in [the figure]. A prolonged constant turn in either direction will result in the false sensation of no turn.
Source: U.S. Army Aeromedical Training Manual, ¶9-20.
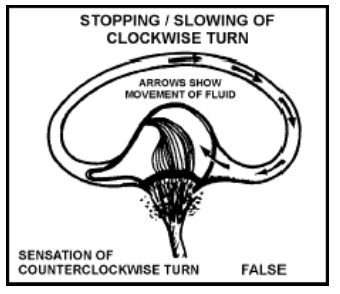
Sensation During Slowing or Stopping of a Clockwise Turn, from U.S. Army Aeromedical Training Manual, Figure 9-12.
When the clockwise rotation of the aircraft slows or stops, the fluid in the canal moves briefly in a clockwise direction. This sends a signal to the brain that is falsely interpreted as body movement in the opposite direction. In an attempt to correct the falsely perceived counterclockwise turn, the pilot may turn the aircraft in the original clockwise direction. [The figure] shows the position of the hair cells—and the resulting false sensation when a clockwise turn is suddenly slowed or stopped.
Source: U.S. Army Aeromedical Training Manual, ¶9-21.
Proprioceptive System
This system reacts to the sensation resulting from pressures on joints, muscles, and skin and from slight changes in the position of internal organs. It is closely associated with the vestibular system and, to a lesser degree, the visual system. Forces act upon the seated pilot in flight. With training and experience, the pilot can easily distinguish the most distinct movements of the aircraft by the pressures of the aircraft seat against the body. The recognition of these movements has led to the term "seat-of-the-pants" flying.
Source: U.S. Army Aeromedical Training Manual, ¶9-22.
4
Illusions leading to spatial disorientation
The book I've used for reference here, Basic Flight Physiology, is quite good. Don't let the illustrations of the light aircraft throw you. The effects are more pronounced in faster aircraft.
The Leans.
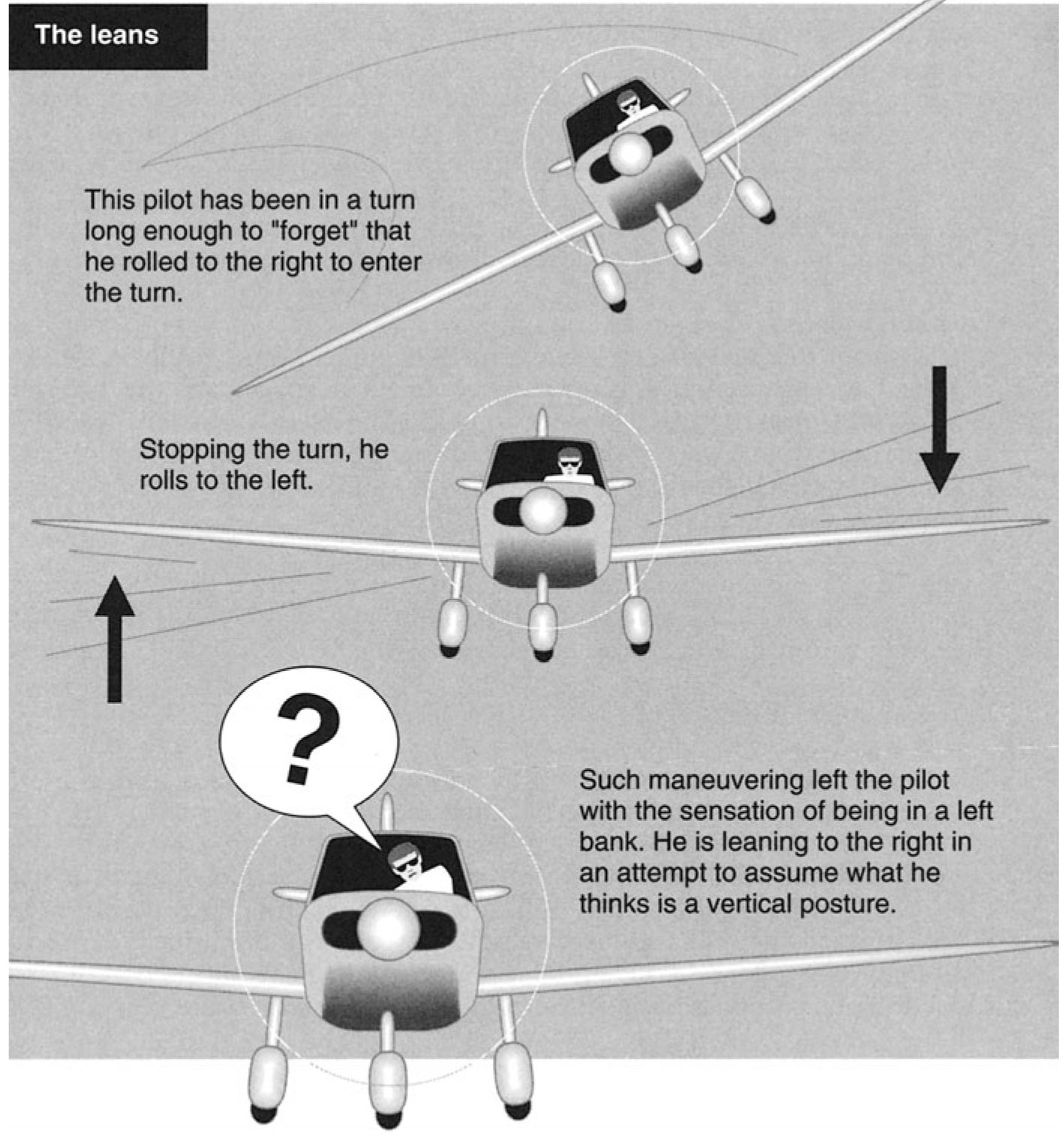
The leans, Reinhart, Figure 8-9
The most common illusion, this occurs when the pilot senses a bank angle when the aircraft is actually in level flight. If she maintains the level attitude of the aircraft (as she should), she will still feel compelled to align her body with the perceived vertical. In doing so, she actually leans in the opposite direction of the perceived turn (Fig. 8-9). The leans can easily occur if the pilot’s attention is removed from the instruments for even a short period of time. For example, if during this distraction the aircraft begins to turn slowly to the right, undetected by the pilot, the canals will respond accordingly. After a period of time in the turn, the brain “forgets” that it is in a turn. When the pilot’s attention is again directed back to the aircraft and instruments and she returns to level flight, the pilot will have a false sense that the aircraft is banking to the left. If she reacts to her senses rather than to the instruments, she will roll the aircraft to the right.
Source: Reinhart, pp. 139 - 140
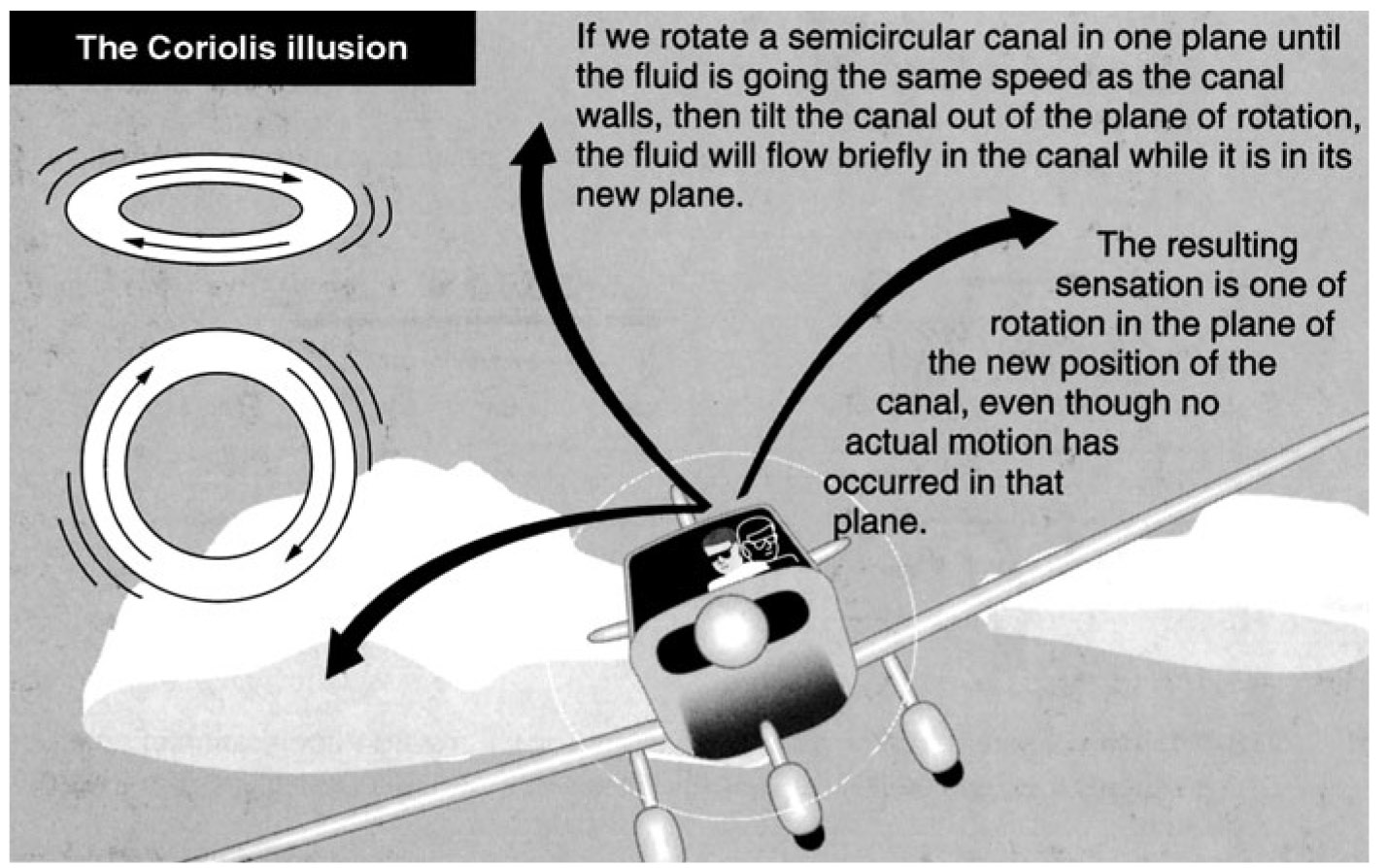
Coriolis illusion, Reinhart, Figure 8-8
- Coriolis. In weather, Coriolis is the circular motion of wind as it passes over a rotating Earth. In physiology, it represents the “tumbling” of our internal gyros, or the semicircular canals (Fig. 8-8). This is the most dangerous illusion because of its often overwhelming sense of disorientation, and it can occur in any phase of flight where some type of climbing or descending turn is initiated. Some pilots described the sensation as being a giant hand taking over the instruments, fighting the pilot’s urge to move the stick.
- When the pilot is straight and level and without acceleration (likewise the aircraft), the fluid in the canals is in equilibrium, not moving. Now, when the aircraft turns and banks, there is an accelerative force on the fluid, which tells the body there is a change. If the pilot stays in that turn and at the same rate, the fluid once again reaches equilibrium of its motion and does not move. In other words, only when there is change does the fluid move and give out a signal. All three planes of the canals are affected to varying degrees, depending on the rate and degree of turn.
- If the turn is coordinated and not abrupt, there is minimal misinformation going to the pilot. If during this maneuver of a turn, the pilot should turn her head in a different direction from the turn, such as downward, one of these geometric planes is disrupted and sends a misleading cue as if the airplane were in a roll or yaw. The Coriolis illusion makes the pilot perceive her airplane to be doing maneuvers that it actually is not doing. This illusion can also be induced in turbulence during a climb or a descending turn. The illusion is caused by the sudden change to the vestibular apparatus.
- Without a visual reference to the horizon, this illusion can overpower the pilot’s recognized need to recover, creating a conflict between the sensation expected in the maneuver and the requirement to control the aircraft against that sensation. It is easier to demonstrate this illusion than to describe it, and this is why unusual attitudes are practiced in flight and why it is recommended that a ride in a Vertigon (simulator that demonstrates Coriolis) be experienced.
Source: Reinhart, pp. 138 - 139
Oculogravic illusion.
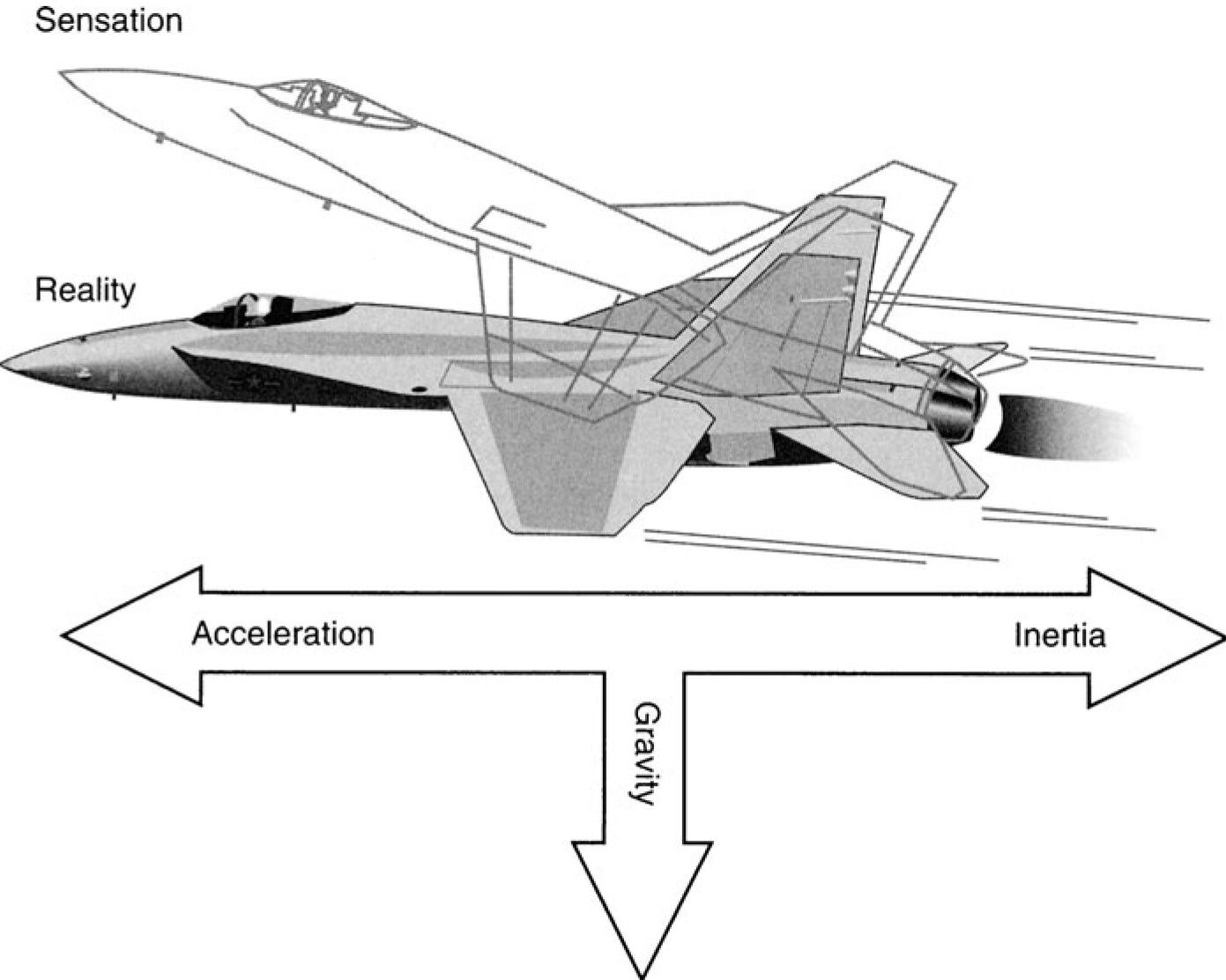
Oculogravic illusion, Reinhart, Figure 8-10
When an aircraft accelerates or decelerates in level flight, the otolith organs sense a nose-high attitude relative to gravity (Fig. 8-10). If that sensation is acted upon by the pilot without cross-checking instruments, he might pitch the aircraft down. Deceleration causes a similar sensation of a nose-low attitude.
Source: Reinhart, p. 140
Graveyard Spiral.
As in other illusions, a pilot in a prolonged coordinated, constant-rate turn, will have the illusion of not turning. During the recovery to level flight, the pilot will experience the sensation of turning in the opposite direction. The disoriented pilot may return the aircraft to its original turn. Because an aircraft tends to lose altitude in turns unless the pilot compensates for the loss in lift, the pilot may notice a loss of altitude. The absence of any sensation of turning creates the illusion of being in a level descent. The pilot may pull back on the controls in an attempt to climb or stop the descent. This action tightens the spiral and increases the loss of altitude; hence, this illusion is referred to as a graveyard spiral. At some point, this could lead to a loss of control by the pilot.
Source: Instrument Flying Handbook, pgs. 1-3 and 1-4
Somatogravic Illusion.
A rapid acceleration, such as experienced during takeoff, stimulates the otolith organs in the same way as tilting the head backwards. This action creates the somatogravic illusion of being in a nose-up attitude, especially in situations without good visual references. The disoriented pilot may push the aircraft into a nose-low or dive attitude. A rapid deceleration by quick reduction of the throttle(s) can have the opposite effect, with the disoriented pilot pulling the aircraft into a nose-up or stall attitude.
Source: Instrument Flying Handbook, pgs. 1-3 and 1-4
I believe pilots who spend a lot of time in the "smooth mode" (trying very hard to keep the airplane experience un-airplane like) are especially susceptible to this illusion. Especially if very tired and distracted, the powerful acceleration given by many modern aircraft can cause senses to tumble. There has been a lot of historical research verifying that this is true, but not enough thought given to survival strategies. That is starting to change. See: Somatogravic Illusion Mitigation Strategies, below.
Inversion Illusion.
An abrupt change from climb to straight-and-level flight can stimulate the otolith organs enough to create the illusion of tumbling backwards, or inversion illusion. The disoriented pilot may push the aircraft abruptly into a nose-low attitude, possibly intensifying this illusion.
Source: Instrument Flying Handbook, pgs. 1-3 and 1-4
Elevator Illusion.
An abrupt upward vertical acceleration, as can occur in an updraft, can stimulate the otolith organs to create the illusion of being in a climb. This is called elevator illusion. The disoriented pilot may push the aircraft into a nose-low attitude. An abrupt downward vertical acceleration, usually in a downdraft, has the opposite effect, with the disoriented pilot pulling the aircraft into a nose-up attitude.
Source: Instrument Flying Handbook, pgs. 1-3 and 1-4
False Horizon.
A sloping cloud formation, an obscured horizon, an aurora Borealis, a dark scene spread with ground lights and stars, and certain geometric patterns of ground lights can provide inaccurate visual information, or false horizon, for aligning the aircraft correctly with the actual horizon. The disoriented pilot may place the aircraft in a dangerous attitude.
Source: Instrument Flying Handbook, pgs. 1-3 and 1-4
Autokinesis.
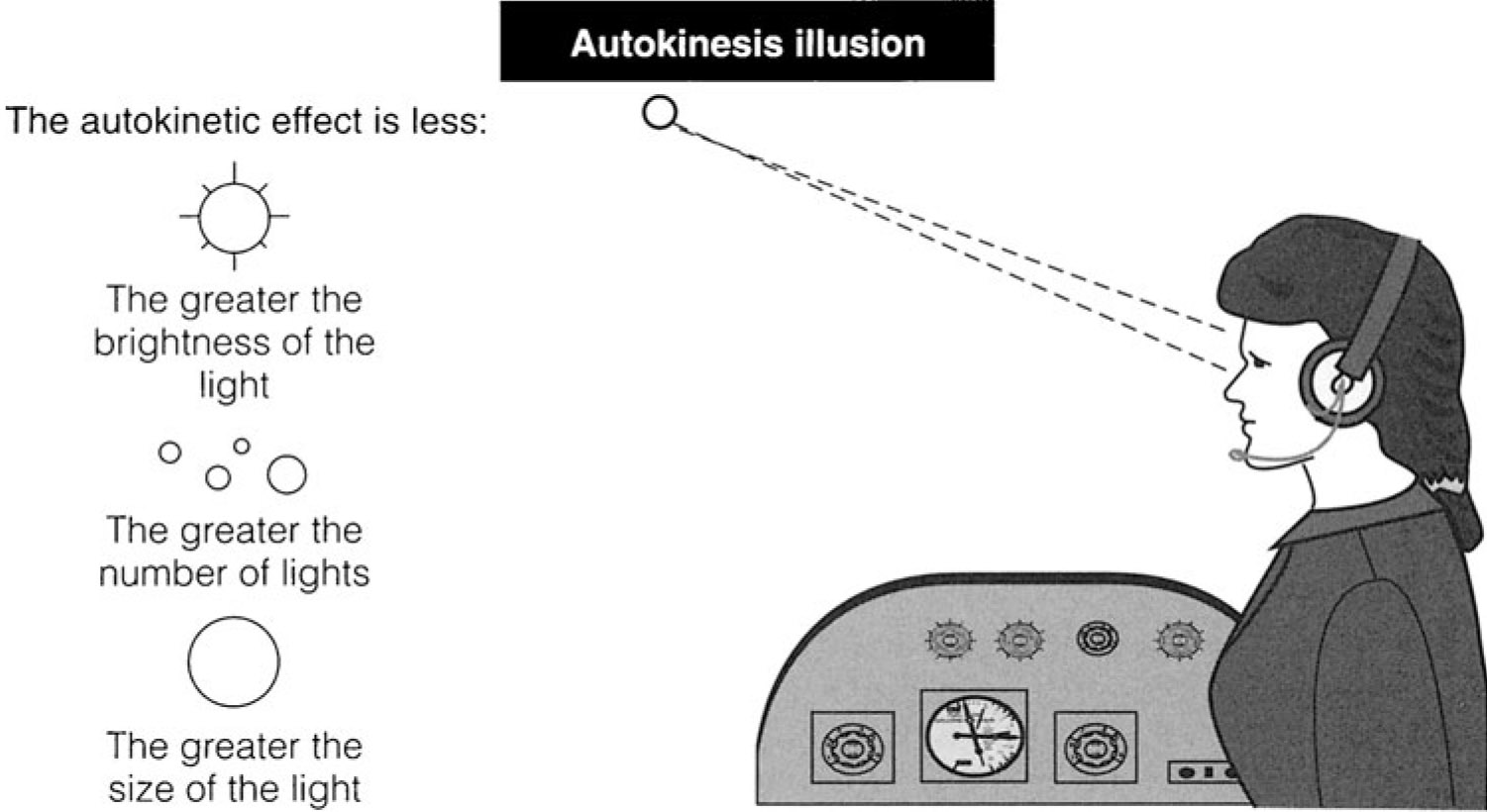
Autokinesis, Reinhart, Figure 8-12
Autokinesis is the perception of false movement when a static source of light is looked at by the pilot for a period of time (minutes) in the dark (Fig. 8-12). This moving reference point (an illusion) could lead the pilot to visually follow it. It is felt that the cause is the brain’s and eyes’ attempts to find some other point of reference in an otherwise featureless visual field. Prevention is a combination of realizing the eyes must focus on other objects at varying distances, not fixating on one target, and basic scanning.
Source: Reinhart, p. 146
5
Somatogravic illusion mitigation strategies
Somatogravic illusions -- the sense that you are pitched up in a climb because of acceleration forces, or pitched down in a descent because of deceleration forces -- have been cited on at least seven large transport aircraft accidents since January 1, 2000, and several before then. The problem is we sometimes lose aircraft for unknown reasons and the lack of tangible evidence places the crash in the "cause unknown" category. For our purposes, we need to assume the somatogravic illusion in many of these accidents to help us come up with survival / mitigation strategies.
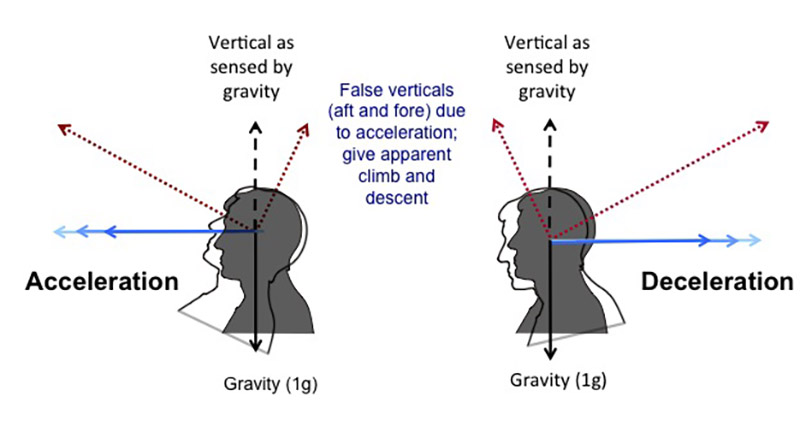
Somatogravic illusion, www.skybrary.aero.
- The somatogravic illusion is an incorrect perception of attitude due to the brain misinterpreting the gravito-inertial acceleration sensed by the vestibular system during prolonged linear acceleration; with reduced, absent or confused visual and proprioceptive information.
- The somatogravic illusion is a particular threat to pilots when accelerating in the anterior/posterior (longitudinal) plane. During the take-off or go-around phases; a pilot lacking visual clues in Instrument Meteorological Conditions (IMC) or at night, may have an impression that they are pitching up and climbing too steeply should any period of longitudinal acceleration last more than about two seconds.
- Descent as a result of this illusion (SGI) has resulted in impact with the surface during these critical phases of flight.
Source: Ludlow, pp. 4 - 5
See the examples, below.
Factors contributing to the somatogravic illusion
- Australia’s BASI [Bureau of Air Safety Investigation] found the range of experience for 18 pilots involved in dark night take off accidents ranged from 189 to 19,006 hours, and the majority of them held instrument ratings, concluding that "low time, inexperienced pilots are no more susceptible to factors in dark-night take-off accidents than more experienced crews”.
- [ . . . ] the age of the pilot was significant in that the older pilots were able to resolve SD conflicts, presumably because of their greater experience.
- Failure to maintain a safe flight path is the cause of all SGI accidents, usually due to an ineffective instrument scan.
- The PM often concentrated more on the management items rather than the aircraft’s flight path and rarely offered warning or advice on any flight path deviations by the PF.
Source: Ludlow, pp. 13 - 14
Factors mitigating the somatogravic illusion
- With more than one pilot on the flight deck, it can be assumed that there is an added level of protection from SGI. This appears to work in some cases: “On a night take off from Runway 07R in Hong Kong with myself acting as PM, we entered cloud at about 1500ft, just as the Flight Mode Annunciator changed to ‘Climb’. The Captain, acting as PF lowered the nose to accelerate to S (Slat retraction) speed, and on passing S, I retracted the Slats as instructed, but noticed the PF had selected a lower nose down attitude than required and was not following the FD commands. The aircraft accelerated rapidly and the PF continued to lower the attitude. When we leveled off, I said “Attitude!” But he continued to push and we started to descend. I then said, “We’re descending”, but with no response. At this point, I took control by selecting an AP [Autopilot] on; the aircraft followed the FD commands and started to climb”
- In one serious incident where SGI was stated as the cause, the PM took control, but only after two warnings from the PM that they were descending which were ignored by the PF who was convinced the aircraft was close to the stall, despite being nose down and at a speed of 245kn (Canada 2009). However, as the trigger for SGI is a physical process, it can affect both pilots equally, as described below: “It was a departure to the North out of Shanghai Pudong airport which involved a track over the sea to depart to the South. It was a clear cloudless night, but very dark. Soon after getting airborne, we were cleared direct to waypoint NINAS some miles down track which involved a right turn through about 160 degrees. With the auto-pilot engaged, this was actioned through the FMC [Flight Management Computer] and at the same time we accelerated to 280kn. During the turn, the wind changed from a tailwind to a much stronger headwind as we climbed, and the auto-pilot pitched the aircraft up to maintain the speed. During this procedure and with the stars in the sky blending in with the lights used by Chinese fishing boats to attract their catch, I got disorientated with a strong sense that the aircraft had pitched up to a very high attitude. I forced myself to believe the attitude indication and with confirmation from the performance instruments, everything was as it should be with an attitude of about 13 degrees up. I checked with my colleague and he had the very same sensation. I know about the somatogravic illusion from my RAF training and immediately recognised it for what it was. Had the AP not been engaged then the automatic response to push forward could have been a very real threat.”
- Somatogravic Illusion accident reports frequently feature manually flown departures and go-arounds (Bahrain 2002, CIS 2006, and CIS 2015-1). Protection afforded by auto-flight systems is lost if the AP is disengaged, either intentionally as in the crash of an A330 at Tripoli in 2010 Libya 2013); or unintentionally as in the case of a B737 crash at Kazan in 2013 where the AP automatically disengaged on initiation of the go-around; a fact not immediately recognised by the crew (CIS 2015-2). Circumstances which requires the aircraft to be flown manually after an automatic approach markedly increases the risk of a SGI event.
- If the crew are so strongly convinced the flight path flown by the auto-flight system is incorrect, they may be tempted to disconnect the AP and fly manually. In this situation, the defences against SGI are lost. Airbus have defined four ‘Golden Rules’, the fourth being “Take action if things do not go as expected” (Owens 2013). This is good advice, except when the pilot has an erroneous perception of the flight path. Despite best intentions, taking control of the aircraft from the auto-flight system could be a costly mistake. It needs to be emphasized that the main function of the auto-flight system is to alleviate high workloads.
Source: Ludlow, pp. 14 - 17
- The auto-pilot should be engaged soon after take- off on departures at night, and/or poor visibility.
- If an approach is conducted at night and/or in poor visibility, the auto-pilot should remain engaged until the required visual references have been established and the landing clearance received.
- If going around at above minima, the manoeuvre should not be rushed and the use of less than full thrust should be considered. If the selection of full thrust is required to initiate the go-around, the selection of lower thrust should be considered as soon as it is safe to do so.
- Consideration should be given to flying go-arounds with the auto-pilot engaged. If the approach was flown manually, an auto-pilot should be engaged as soon as possible.
- If the approach is flown with the auto-pilot engaged, it should not be disengaged for a go-around.
- The Pilot Monitoring should concentrate on monitoring the flight-path in the climb and go-around phases.
Source: Ludlow, pp. 18
6
Examples of spatial disorientation
22 July 1973 — Pan American World Airways 816 (Boeing 707-321B) — Taking off on a moonless night from the island of Paprette, the horizon was not visible. The captain appeared to enter a descending left turn right after takeoff, right into the ocean. Of 79 people on board, only one survived.
23 August 2000 — Gulf Air GF072 (Airbus A320-212) — The captain appeared to suffer from spatial disorientation after going around from an approach that had to be balked because he was too fast and too high. During the go around, at about 1,000 feet, the captain pitched down 15° and impacted the sea doing 280 knots. The first officer did not callout these deviations. All 143 persons on board were killed.
3 January 2004 — Flash Airlines 604 (Boeing 737-3Q8) — The captain experienced vertigo right after takeoff and disengaged the autopilot because the instrument indications did not agree with his senses, he expressed a degree of confusion when the first officer announced he was in a right turn. The first officer did not make any assertive moves to take control of the aircraft or forcefully correct the captain's actions. They crashed into the sea in a descending right turn, killing all 148 persons on board.
3 May 2006 — Armavia 967 (Airbus A320-211) — The captain "made nose down control inputs due to the loss of pitch and roll awareness" while aborting an instrument approach. The copilot failed to monitor the approach properly and neither reacted correctly to EGPWS warnings. All 113 people on board were killed.
16 October 2013 — Lao 301 (ATR 72-212A) — The crew flew an instrument approach with the altitude select feature of their autopilot set to the MDA set to the nearest 100 feet, but low. The crew disconnected the autopilot and initiated the missed approach but the go around vertical mode immediately switched to altitude hold. The pilot began a right turn (which was not part of the missed approach procedure) and began losing altitude. The captain pitched up to 33°, and then down again until impact with the river. All 49 persons on board were killed. Somatogravi illusions were listed as a possible cause.
17 November 2013 — Tatarstan U9363 (Boeing 737-53A — The captain didn't understand that the Take Off / Go Around function of his aircraft would disengage the autopilot), the crew advanced the power, retracted the flaps and gear, but made no pitch inputs of their own. The aircraft pitched up to 25° at which time the captain pitched down aggressively to about 75° nose low, reaching about 5,000 fpm before impacting the terrain, killing all 50 persons on board. The captain's pitch down was thought to be as a result of spatial orientation.
References
(Source material)
Army Field Manual 3-04.301, Aeromedical Training for Flight Personnel, 29 September 2000z
Air Force Manual 51-37, Instrument Flying, 1 December 1976
FAA-H-8083-15, Instrument Flying Handbook, U.S. Department of Transportation, Flight Standards Service, 2001.
Ludlow, Simon. Reducing the Threat of the Somatogravic Illusion, Flight Safety Foundation, 2016.
Reinhart, Richard, M.D., "Basic Flight Physiology," Third Edition, McGraw Hill, 2008.